The LFP 4680 Battery Cell // + LFP with Tesla Silicon
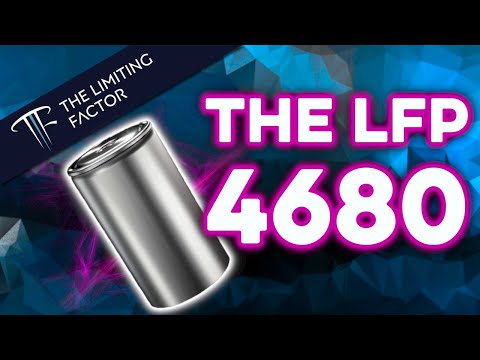
Welcome back everyone. I'm Jordan Giesige and this is The Limiting Factor. I made this video before the Q4 2021 earnings call. And at that earnings call Drew and Elon did make some comments in relation to 4680s with LFP. So, I thought before we kick into the video, it would be good for me to give my perspective on what was said at that earnings call. Let's start by working through the comments that Drew and Elon made, and then we'll move into the video. Drew said, quote, “To the question about, should everything be 4680?
It doesn't have to be. In the end, it's about cost competitiveness and scalability of manufacturing.” So my thoughts on that are: This gels with what I'm saying. Throughout the video I talk about there's…you could go with a 4680 or prismatic, but there's trade off decisions that you have to make. Now it's also worth noting that Drew's comments were prompted by Pierre Ferragu’s question. And his question was focused on outside self-supply. So, Drew's comments don't necessarily
apply to Tesla's in-house 4680 or whatever form factor that they decide to use for LFP. Drew continued on by saying quote, “There are just some physics-based differences in what happens in certain corner cases that would drive different form factors. And we just have to be cognizant of that and design to that.” End quote. As I said a moment ago, different form factors have positives and negatives. Just like you can change the thickness of the electrode and a battery, so, or change the electrolyte mix depending on if you're using those battery cells for energy storage or high power applications like vehicles. So, what drew
might be suggesting here is that they might use different form factors for different use cases, such as mobility, like vehicles or energy storage. Uh, as I say in this video, uh, a 4680 form factor, because it's smaller, it's easier to thermally regulate. So, they might use 4680s for their vehicles and a prismatic battery cells for energy storage.
And in fact, they, they still might be exploring this. One interesting thing to note is that it might actually change over time. Now, overall, as I say, in the video, my preference is for Tesla to use the 4680 for everything, just to keep things simple, but they do have these avenues open to them. So, with that, let's move on to Elon's comment, which is, “Yeah, we don't use the 4680 at all for the Iron based cells.”
This is something we already know from, from my perspective, this was a present tense comment by Elon, and it speaks directly to what Pierre Ferragu’s question was, which is, “Our outside suppliers all going to switch to a 4680 form factor for all their battery cells.” And CATL already uses a prismatic LFP cell for the battery cells that supplying to Tesla. So, there's no reason for them to switch that up if that's hitting costs and scalability requirements. Now, that doesn't preclude other battery cells or future manufacturing lines from using the 4680 form factor for LFP or nickel-based cells. It's just that, uh, it doesn't have to be used for everything. And they're currently not using the 4680 for the cells they get from, for instance, CATL Now with all that out of the way, let's kick into the video. There are two questions I’m consistently
asked about when it comes to the topic of LFP: “Can Tesla produce LFP in a 4680 battery cell” and “Will Tesla produce LFP in a 4680 battery cell?” The answer to the first question is easy: Yes, all the technologies unveiled at Battery Day are compatible with LFP, so Tesla can produce an LFP 4680. The question of will they produce and LFP 4680 is more complex, but I think the answer is also yes. This video will cover how I came to that conclusion, the benefits and drawbacks of using a 4680 form factor, a roadmap for energy density improvements, and why I think LFP will become the dominant lithium chemistry. Before we begin, a special thanks to my Patreon supporters and YouTube members. This is the support that gives me the freedom to avoid chasing the algorithm and sponsors. As always, the links for support are in the description.
First, for those who aren’t familiar with what LFP means, it’s simply the abbreviation of Lithium Ferro-Phosphate. Ferro means iron, so what we’re talking about is an iron based cathode. LFP’s primary benefits are that it’s cheap, durable, and easy to scale. But it has a catch. LFP batteries have low volumetric energy density compared to commonly used Nickel based chemistries, meaning they take up a lot of space. To counteract the low volumetric energy density, LFP batteries are typically large and use a rectangular format known as prismatic. Prismatic battery cells maximise the rectangular shaped volume under the vehicle so manufacturers can squeeze in more energy. This is as opposed to cylindrical cells which have a maximum packing efficiency of about 78% in a rectanguloid or cuboid box. This poses a
challenge for Tesla because they’ve chosen a cylindrical format for their in-house battery cell production. The dimensions are 46 by 80mm and it’s why they named the format the 4680. So, the question naturally arises as to whether Tesla will manufacture LFP batteries in a 4680 format given that it has an energy density handicap versus a prismatic cell. CATL LFP Model 3s out of China are now getting about 273 miles of range with a 60 kWh pack. This is with a prismatic battery cell, which has a theoretical packing density of 100%. Can Tesla hit 273 miles of range with a cylindrical format that has a 78% theoretical packing density? First, although the theoretical packing efficiency of prismatic battery cells is a 100%, in reality, manufacturers like CATL only hit about 42% due to the steel bracing and other packaging. Second, cylindrical battery packs like Tesla’s achieve a 33% packing density. However,
that battery pack was designed about 5 years ago and also uses steel bracing, more fireproofing, and other packaging such as modules. That is, just by eyeballing it, it looks to me that if Tesla can strip out the steel bracing and some of the other excess packaging, they should be able to match the energy density of a CATL LFP battery pack. As we know, stripping out the steel bracing and excess packaging is exactly what Tesla is attempting to do with the structural battery pack that they unveiled at battery day. Let’s run the numbers on a structural pack with LFP. Sandy Munro indicated that a structural battery pack using the 4680 could fit 960 cells under a Model 3. To double check Sandy’s claim, I checked with Alex Tourville, who’s done a lot of work and given some great presentations covering 4680 cell and pack design on the Official X Pod YouTube channel and on the Callin app. He indicated that the maximum pack size would depend on safety margin and pack orientation and could be anywhere between 912 and 1064 cells for a Model 3. What that in mind, I think Sandy’s
guess of cells is a good middle ground. How much energy will each of those cells contain? The 2170 battery cell used in the Model 3 and Y stores roughly 18 Wh of energy. If a 4680 cell stores 5x the energy due to its larger volume that would mean a 90 Wh battery cell.
Again, I’m being conservative here. The 4680 has nearly 5.5 times the volume of a 2170 cell rather than 5x the volume. Let’s convert that 90 Wh Nickel cell into a comparable LFP battery cell. In terms of volumetric energy density, Tesla’s Nickel based 2170 battery cells are 720 Wh/l while BYD and CATL LFP are maxing out at 450 Wh/l. That is, LFP has roughly 63% of the volumetric energy density of the 2170 nickel battery cell. 63% of a 90 Wh battery cell is 56 Wh per battery cell. 56 Wh times 960 battery cells is 54 kWh. 54 kWh is roughly the same size as the pack on
the 2021 standard range plus Model 3 LFP vehicles from China, which achieved 253 miles of range. So, the answer to the question “Can Tesla make a 4680 cell using LFP?” gets a yes from me, because it looks like they can make a battery pack that satisfies Tesla’s 250 mile minimum range requirement. Furthermore, I was conservative in most of the estimate steps, which leads me to believe that Tesla could overshoot 250 miles of range with LFP in a 4680 form factor. More on that in a moment. Is there any indication from Tesla that they’ll use the 4680 for LFP? Yes…ish. Tesla unveiled the LFP chemistry in the same presentation that they
unveiled the 4680 battery cell. Then, at the end of the presentation, Drew Baglino said that the benefits of Tesla’s structural battery apply to an iron based cathode the same way they apply to a nickel based cathode. That is, Tesla didn’t explicitly say they’d use the LFP in a 4680, but my view is that this was implied because the structural battery uses 4680s. But why does it matter whether Tesla can use LFP in the 4680 form factor? If Tesla didn’t use the 4680 for their LFP battery cells, it would require the design of new battery cells and packs as well as the manufacturing lines to make those battery cells and packs. That is, using different form factors for different chemistries would add complexity to Tesla’s cell manufacturing operations, which runs counter to Tesla’s tendency to reduce manufacturing complexity. Furthermore, those who don’t think that the 4680 form factor’s viable for LFP and want to see Tesla do prismatic LFP may be placing too much emphasis on energy density. Although prismatic battery cells do offer higher theoretical energy density
than cylindrical cells due to their better packing efficiency, they also come with a draw back. They tend to have low surface area for a given volume. This makes regulating the temperature of prismatic battery cells more difficult for both high and low temperature performance. LFP is robust to high temperatures, but as LFP chemistries become more energy dense and the prismatic cells are packed more tightly, prismatic battery cells may hit a thermal limit. That is, there may come a point when prismatic cells reach a high enough energy density and packing density that a smaller battery cell becomes necessary to prevent degradation issues from trapped heat.
As for low temperatures, in my LFP science video I showed that LFP battery cells perform best when pre-heated. 4680 cells will have a smaller internal volume than prismatic battery cells, which may allow for quicker pre-heating of the 4680 battery cell in colder climates. What if I’m wrong, and Tesla can’t make the 4680 work with LFP. Could Tesla make a prismatic LFP battery cell? Absolutely. As I said earlier,
it would just require further investment into R&D and separate battery cell lines. The question is, would that be the best use of Tesla’s engineering resources and would they be able to recuperate the R&D expenses. Those are two questions I don’t have an answer to and they’d be tightly linked. Let’s press on and look at the full potential of a 4680 cell using LFP. As I said earlier, 250 miles of range with a Tesla LFP 4680 cell is the base case. If we take into account that I was
conservative and that Tesla might have a trick up their sleeves with the thick DBE cathodes I talked about in the secret sauce video, they might be able to get that to 275-300 miles of range. What about beyond 300 miles of range? Let’s look at high loaded silicon anodes. By a high loaded silicon anode, I mean adding 5-30% Silicon to the graphite normally used in a lithium ion battery anode. This is versus the 0-5% silicon commonly used in graphite mixes today. To
keep things simple, I’ll refer to the 5-30% high loaded silicon anode as a high silicon anode. I’ve covered silicon doping of LFP a couple times in the past, but my view continues to develop on the topic. My original view was that Silicon would handicap one of the biggest benefits of LFP, which is high cycle life. LFP is good for 3000 cycles and beyond, which makes it perfect for commercial
applications like energy storage and Robotaxis. Meanwhile, Silicon struggles to hit 1000 cycles in a battery anode due to issues related to the expansion and contraction of the silicon. That is, why kneecap the cycle life of LFP for additional energy density when the primary use case would be Robotaxis and energy storage, where cycle life plays a large role in the economics. Furthermore, LFP battery cells are, so far, unprofitable to recycle because the value of the iron in LFP batteries is low. With Nickel based battery cells recycling makes more sense because Nickel is more than an order of magnitude more expensive on the open market than iron.
Because the recycle value of LFP is so low, my view was that the cells would be trashed at the end of life rather than recycled. It that were the case, it would make sense to leave out the silicon to maximise cycle life, which would reduce the carbon impact of a single life cell. However, for several reasons, I’m now getting more bullish on Silicon for LFP batteries. First, on the recycling front, JB Straubel is giving recycling a big push with Redwood Materials, and I think his involvement will accelerate scaling and therefore improve the economics of recycling LFP batteries. By the time LFP batteries start
coming off the road in about 10-15 years, recycling LFP batteries might make economic sense. Second, somehow, I missed a bombshell comment from Drew at Battery Day in the Q&A section. Drew stated that Silicon is a key part of the roadmap for iron based cells. That is, Tesla has stated they plan to use Silicon in their in-house LFP battery chemistry using the 4680. I see two potential technology pathways here: First, a single chemistry pathway where high silicon anodes are used in all of Tesla’s in house LFP battery cells. This would happen if they’re able to achieve 3000+ cycles with Tesla silicon, which would match the 3000+ cycle life that LFP is capable of. If Tesla silicon achieves anything less than around 3000 cycles,
it would limit the cycle life of the entire battery cell to the cycle life of the silicon, which brings us to the second pathway. If Tesla hasn’t fully cracked the Silicon code, they may manufacture two different LFP chemistries. First, a workhorse LFP chemistry using no silicon that offers 3000+ cycles for energy storage products. Weight and volume aren’t a primary concern for energy storage products and the silicon wouldn’t be needed. Second, a mobility LFP chemistry using a high silicon anode that offers around 1,000 cycles for vehicles.
Silicon would reduce the weight of battery cells and therefore the weight of Tesla battery packs, which would increase range, but there would be a hit to cycle life. However, 1000 cycles would still be acceptable. 1000 cycles would mean around a 300,000 mile service life on a vehicle with more than 300 miles of range. A 300 mile range vehicle should be no problem for LFP if it has a high silicon anode. More on that in a moment. Will Tesla take path A or path B? My guess is that Tesla hasn’t fully mastered the durable silicon required for 3000+ cycles because it’s such a difficult problem to solve. Meaning I think they’ll take path B and pair their LFP cathode with two different anode options. This is much the same way slightly different electrolyte
formulations and electrode thicknesses are often used in the battery industry for high power, high energy, or high cycle life cells. If Tesla does have high cycle life Silicon, I’ll be gobsmacked and be happy to be wrong. Going from the 0% Silicon used in current LFP chemistries to 5-30% Silicon with high cycle life is like skipping the amphibian stage in evolution and going directly from fish to lizard. Regardless, the key takeaway here is that
Drew indicated that they plan to use Tesla Silicon in Tesla 4680 LFP batteries, which will expand the scope of use cases for LFP and open the door to long range LFP based vehicles. How much would high silicon anodes increase range for LFP based vehicles? On our base case 250 mile range vehicle, a high Silicon anode would increase range by 20% to 300 miles of range. However, there are three things worth considering along with that estimate: First, the range boost probably wouldn’t happen all at once. Tesla would likely
titrate Silicon into their LFP chemistries over the course of a few years. Second, the 250 mile base range was conservative. If Tesla can hit 270 miles without silicon, they could eventually hit 325 miles of range or more in the mid-2020s with a high silicon anode. Third, LFP’s already poised for dominance in energy storage products because of its high cycle life and low cost AND it’s already making great inroads into short range and mid-range vehicle segments. If Tesla was able to achieve 325+ miles of range with a high silicon LFP
chemistry, LFP would gain dominance in both grid and automotive use cases. With that in mind, it quickly becomes clear why Elon has said that the ratio of iron to nickel based battery cells will be 3:1 or more. At Battery Day, Tesla indicated that the energy storage market is worth 10 Terawatt hours and the transportation market is worth 10 terawatt hours.
A 3:1 ratio would be 15 terawatts of LFP chemistries and 5 terawatts of Nickel chemistries. In 2021 about 100 GWh of LFP battery cells were produced, which means the market for LFP could grow by 150x in the next 15 years or so. It’s worth noting that I’m purposely leaving Sodium ion batteries out here, which’ll be covered in a separate video.
Earlier I said that LFP with a high Silicon anode could easily hit 300 miles of range but 325 miles or more was certainly possible. I also said that my definition of a high silicon anode was 5-30%. Clearly, LFP has even more runway beyond 325 miles. On Twitter, Matt Lacey did some rough calculations for LFP when paired with advanced anodes and electrolytes. At the cell level, with 25% Silicon, the energy density was around 500 Wh/l. With 100% Silicon or lithium metal, it was around 600 Wh/l. For reference, Tesla’s Nickel based 2170 chemistry used in the Model 3 and Y is 720 Wh/l. However, these numbers are all at the cell level. Typically, LFP batteries require less packaging material because it’s a safer chemistry than Nickel. Plus, we need to factor in that by the time we see these advanced chemistries
in the late 2020s, there’ll be further efficiency improvements throughout the power train. With all that in mind, my view is that an LFP 4680 battery pack could eventually provide 400 miles of range. As a side note, with other LFP form factors such as the BYD Blade, it could be possible to hit 400 miles of range without 100% Silicon anode or lithium metal. This is because prismatic based battery packs have a higher theoretical energy density than cylindrical based packs. However, Tesla’s efficiency is several years ahead of Chinese manufacturers.
With so many variables in play, If Tesla does manufacture LFP based 4680s, it’ll be an interesting case study to watch the competition between Chinese made prismatic LFP based vehicles vs Tesla made cylindrical LFP based vehicles. With all that said, form factor may be an academic point. First, Tesla can always develop an in house prismatic LFP battery cell. From a customer perspective, does it really matter whether the battery cell looks like a SPAM can or a Campbell’s Soup can? Second, Tesla’s already purchasing prismatic LFP battery cells from CATL and will likely be purchasing them from BYD. That is, even if Tesla does use the 4680 for in house LFP cells, many of their vehicles will be using prismatic LFP and it’s unlikely Tesla would allow all those vehicles to have much variability in their performance characteristics. So again, the difference would be transparent to customers. Are there any barriers to Tesla adopting LFP? None that I can see besides developing and qualifying a new LFP cathode material. As I covered in the LFP Patents video,
the patents should be expired by April of 2022. My guess is that Tesla could deploy LFP in 2023 to 2024 after they have their first Nickel based production lines up in Berlin and Austin. Are there any barriers for other auto manufacturers looking to adopt LFP for their electrification efforts? For western automakers that are expected to offer mid to long range vehicles, definitely. Every other automaker has an efficiency about 20% less than Tesla’s, and in most cases that’s being generous. Instead of 273 miles of range from an LFP pack, they’d get about 218 miles of range. That 20% efficiency handicap could spell disaster for some of these companies because they’ll be forced to use Nickel based battery chemistries instead of LFP. Nickel is difficult to source and supply shortages are
expected throughout the decade, meaning shortages of Nickel based battery cells. Shortages of Nickel based battery cells may mean that if a manufacturer can’t adapt and use an LFP based battery pack, their electrification efforts may fail because they simply won’t have the batteries they need to make vehicles. Furthermore, LFP battery packs are 20% cheaper than Nickel based battery packs, which gives manufacturers who can use LFP battery packs operational head room.
In short, electric vehicle efficiency may determine whether some EV makers survive or go bankrupt in the 2020s. Tesla’s been working on their core vehicle efficiency for about 15 years, which allows them to use fewer battery cells in each vehicle, increases margins, and opens up more chemistry options and therefore a larger cell supply to ramp vehicle production. With regards to Chinese manufacturers, their domestic market isn’t as demanding for range and I expect the efficiency of Chinese vehicles to increase rapidly, which’ll make them competitive in North American and European markets. That is, Tesla and Chinese manufacturers seem to be the best positioned with LFP. Let’s wrap things up. The Tesla 4680 cell is compatible with an LFP battery chemistry and should provide a range similar to the current LFP variant of the standard range plus model 3, at around 250-260 miles of range. This is true even with a conservative estimate and before factoring in potential energy density improvements from silicon. 250-260 miles o f range is a minimum.
In the next few years, Tesla could achieve 300 miles of range with LFP in the Model 3 with a moderate loading of Silicon, 350 miles of range in the mid-2020s with a high silicon loading, and eventually 400 miles of range fully evolved with a 100% silicon anode or lithium metal anode. Prismatic battery cell formats do have some first principles advantages but, as Tesla indicated at Battery Day, if more range is needed, they can use one of their other chemistries. Nickel will remain the premium chemistry for range and performance and LFP will be the work horse. Why develop an entirely new cell and pack architecture if you can just change your cathode coating mixture, at any of your factories, at any time, from a Nickel coating to an LFP coating, and vice versa.
It’s a little more complicated than that, but that’s the general idea. Changing the coating mixture versus tens, possibly hundreds of millions of dollars of R&D expense. From a technology standpoint, a prismatic LFP cell could leverage most of the technology unveiled at battery day, but it still wouldn’t be cheap to develop. I’m not sure if the tabless electrode could be used, but it might allow Tesla to make a large prismatic cell with good thermal regulation.
Regardless, if a prismatic form factor allows Tesla to recuperate the R&D expenses, they’ll go prismatic for in house LFP. I don’t imagine the savings would be large, but every penny counts. What about cost? How cheap will Tesla LFP battery cells be? As discussed in the BYD blade battery video, prior to the current bout of inflation, a CATL LFP battery cell cost about $88/kWh at the pack level whereas the BYD blade battery pack costs about $65/kWh. Tesla’s cost would be at or below the cost of the BYD blade battery pack when they hit scale. This is because Tesla will have the advantage of a higher throughput on their battery cell lines and won’t be paying a margin to a third party for the cells they produce in house.
That is, I’m expecting below $50 per kilowatt hour at the pack level around 2025, possibly as low as $40 per kilowatt hour at the pack level. That comes with a big caveat - that Tesla can get their materials cheaply. Battery materials are currently running short and there’s inflation in the market. What’s the price floor for LFP batteries? My guess is perhaps as low as $20-25 per kilowatt hour at the pack level by the mid twenty thirties. It would take an
entire video and some cost modelling to prove that out, and there would be some significant potential error bars, but I think it’s doable. Regardless, LFP batteries are inherently cheaper, and the materials are more widely available. That is, the cost and scalability of LFP will make electric vehicles affordable and widely available. For those manufacturers that lag on efficiency,
it may be more difficult to use a lower energy density chemistry like LFP, and it’ll make their scaling efforts more difficult. Tesla’s been preparing for this moment for decade and has the efficiency and adaptability to incorporate LFP into their line up rapidly, where it will eventually displace Nickel as their go-to battery chemistry. In the next video of the LFP series, I’ll wrap up the entire LFP series with a summary video. If you enjoyed this video, please consider supporting me on Patreon with the link at the end of the video or as a YouTube member. You can find the details in the description, and I look forward to hearing from you. A special thanks to Mike Gabrys, Dave Fitzhugh, James (The Illustrious) Douma, Asbjørn S. Tunheim, Stefan Sommer,
and Chan Nguyen for your generous support of the channel, my YouTube members, and all the other patrons listed in the credits. I appreciate all your support, and thanks for tuning in.
2022-02-10 13:15