Why does Starship belly flop?
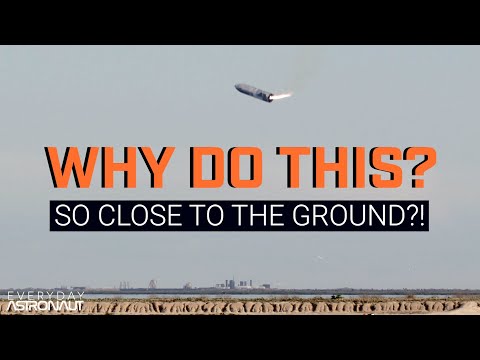
- Hi, it's me, Tim Dodd, the everyday astronaut. SpaceX is trying some pretty, crazy things with their Starship rocket. But perhaps there's nothing more crazy than letting your nine meter wide, 50 meter tall rocket fall out of the sky, belly first. And then try to light your engines basically very last moment, in order to go from horizontal to vertical, to hopefully land softly. Now, the reason they're doing this is so they can make their terminal velocity as slow as possible, so the rocket engines don't have to do nearly as much work slowing the rocket down. Now, in order to understand what exactly that all means, there's a ton of physics lessons to unpack here.
And seeing all of these spectacular failures, has brought up a lot of really good questions from you guys. But perhaps there's no bigger question than why. Why are they doing that belly flop maneuver? Why are they going from belly first and then flipping to tail down? And why are they doing that maneuver so late? Why don't they just start that landing and maneuver just a little bit earlier, to make sure there's enough time to make corrections, if something doesn't go right? But we're also going to look at what other options and constraints and variables SpaceX has with running on different number of engines. Is running on more engines better? Is starting that landing burn earlier better? And can they use parachutes as a backup, if something goes wrong? And of course, we'll answer the ultimate question. Will this belly flop maneuver ever be safe enough for humans? I mean, could you even survive the G-forces of that crazy flip? Should SpaceX just scrap this whole idea and go back to landing at more like a Falcon 9, which has proven to be highly successful.
So today we're going to cover the wonders of terminal velocity, thrust to weight ratio, gravity drag and engine throttling, to see if we can figure out why SpaceX is pursuing such a crazy landing maneuver. And if we even think that's a good idea or if maybe they should just go back to the drawing board. Let's get started. (bright sound) (upbeat music) Now, right off the bat in case you haven't watched it already, you might want to watch my complete guide to Starship, as we actually go over a lot of things that are going to be talked about in this video. So it might be helpful if you haven't seen that. Or if you have other questions about Starship in general after you watched this video, give that one a watch, it'll likely help you find some answers.
But the point of this video is to explain the physics behind the rocket science, not to try and speculate but we're going to help you understand why. So when SpaceX does make changes, which they inevitably will, you'll have some grasp on why they may have made those decisions. But this is a long video. And like all my long videos, we've got some timestamps for you guys for easy watching or quick skipping to these certain sections you might want to see. We also have the YouTube play bar broken up in those same sections. And we have an article version of this video up on our website at everydayastronaut.com.
The link's in the description so that you can quickly search for certain topics. (bright music) Starship is doing something completely unique here, with this landing maneuver. So, here is how it works or I guess how it's supposed to work. Starship reenters and falls out of the sky belly first, to scrub off as much velocity as possible while falling. At about 500m in altitude, it lights up it's Raptor engines, gimbals them full tilt, folds in the rear flaps and swings from horizontal to vertical, so it can land tail down. By having the rear fins or Aft fins or as I might call them Alanna Ron's tuck in and by keeping the nose fins extended, it makes it so the nose has much more drag and will want to point up and the tail will want to fall down, which will help to aid in the rotation.
In later versions of Starship, we'll likely see powerful hot gas thrusters, that could aid in this flip. But as of the making of this video, they've been using purely cold gas thrusters, basically off of a Falcon 9. But they only use that to get into the belly flop for this testing period but they don't actually aid in the flip itself.
Because the rocket is lighting its engines while horizontal, it needs to pull propellant from special tanks called header tanks, so that the engines don't suck up gas bubbles. Because of course the main drain valves on a rocket's propellant tank are usually at the bottom of the tank which for a normal rocket is typically oriented point end up , flaming end down. While Starship is falling through the atmosphere, the propellant will settle on the belly side of the rocket, otherwise known as the Windward side. Now Starship has these special header tanks, that are essentially just reserve propellant that are nearly full and have their drain valve at a little bit of an angle, which is perfect for the landing maneuver. But also because the rocket lights its engines while it's horizontal, it'll inject a lot of horizontal velocity and then intentionally over rotate, all the way beyond vertical in the opposite direction, to cancel out that horizontal velocity.
Once it begins to rotate back to vertical, it tucks in its top flaps and will precisely control itself down to a nice soft touchdown, much more like a Falcon 9 booster landing. So for now I think we need to answer the most burning question, why. Why are they even doing this absurd maneuver and not just landing like a Falcon 9, since that obviously works, really well? (bright music) - Okay, so let's start with the belly flop maneuver.
Well, like we mentioned at the top of this video and in our complete guide to Starship, it's all about scrubbing off as much velocity as possible. Let the atmosphere do as much work as it can, essentially for free, no propellant necessary. But one of the biggest reasons that Starship will be coming in belly first is actually to control peak temperatures and orientation of Starship during orbital re-entry. Which is a huge deal, especially when trying to land at a precise location on Earth or Mars.
Now we're not going to be focusing on that orbital re-entry portion in today's video because I've covered it a bit in some other videos. So let's try and figure out why Starship continues to belly flop once it's in the lower portions of the atmosphere, when it could easily straighten itself out at a higher altitude and land more like a Falcon 9. So the key here is getting your terminal velocity as slow as possible.
Terminal velocity is the maximum velocity an object reaches while falling through a fluid such as air. Yes, air is a fluid. It's when the downward force of gravity equals the force of drag. So the more drag an object has, the slower it's terminal velocity.
It's the same reason why a feather will fall slower than a hammer. Well, at least here in Earth's atmosphere. - And I'll drop the two of them here and hopefully they'll hit the ground at the same time. How about that? - Now, of course, terminal velocity actually changes based on local conditions. As the atmosphere gets thicker and thicker, drag gets higher, so the terminal velocity is slower and slower.
But as long as your drag is equal to gravity, you are at terminal velocity, even when that velocity number is changing. Just like skydiving, if you fall belly first, your terminal velocity is at a minimum and you can spend as much time falling as possible, while also maintaining a lot of control with your arms and legs. Now, imagine if you were skydiving feet or head first, you'd fall substantially faster because the oncoming air has much less surface area to hit and slow you down, compared to belly first. So the atmosphere can't do as much work and your terminal velocity would be much higher. And if we just look at the belly of Starship versus looking at the bottom of Starship, you can see just how much more cross section there is.
There's approximately 545 square meters of surface area on the belly side but only about 70 square meters of surface area on the base of the rocket. That's 7.8 times more surface area to help slow the rocket down. Of course the mass would be the same, no matter the orientation. So if you have 7.8 times more surface area, there's a lot more surface for all the air to push against.
All things considered equal, the terminal velocity will be substantially slower. Now granted the actual coefficient of drag is vastly different between the concave engine section and the rounded cylindrical body of the rocket. But even so the terminal velocity is substantially slower falling belly first. But perhaps one of the most fun things about using Starship as an example of terminal velocity is they can actually change its terminal velocity, based on how deployed all of its flaps are. While belly flopping, if Starship deployed all of its flaps out more, it's terminal velocity would be slower because it has more drag.
If it were to tuck its flaps in a little more, it's drag would decrease and it would fall faster through the atmosphere with a higher terminal velocity. Now in general SpaceX doesn't want the flaps to be maxed out, so either all the way open or all the way closed. Because they would be incapable of making further adjustments to maintain orientation and control. So they will likely want to be roughly in the middle remaining relatively neutral. And we can actually see the difference of velocity in the telemetry of Starship versus a Falcon 9.
Let's take a look at Declan Murphy's awesome Flight Club data of the two. This is a highly accurate simulation of Starship SN8's flight profile and the NROL- 108 Falcon 9 mission. For these initial medium altitude tests like we've been seeing, Starship free falls a bit after it gets into the belly flop.
It slows down as it gets lower and lower and into thicker parts of the atmosphere, until it slows all the way down to only about 90 meters per second, before lighting its engines for the flip and landing burn. Now, of course, if Starship was falling from a higher altitude or coming back in from orbit, there would be a higher peak velocity. But once it gets into low altitudes and in thicker atmosphere, it should reach terminal velocity, which is very low by the time it lights its engines. And that is great. So now let's look at the Falcon 9. Notice that after the entry burn, the Falcon 9 is back in freefall.
It actually speeds up a little after engine shutdown because the atmosphere is still pretty thin at 40 kilometers. But then it slows down more and more and the more the rocket gets into the lower parts of the atmosphere, the slower it gets. And don't forget, Starship won't ever need to do any kind of re-entry burn after its deorbit burn at all, because it will use its heat shield to protect itself during re-entry and the entire broad side of the vehicle to slow down.
So despite coming in at orbital speeds, and not just suborbital speeds like the Falcon 9, it will only have to do one landing burn. And that right there is a substantial difference. But even for the final landing burn, staying in the belly flop as long as possible, still pays off.
Because notice right before the Falcon 9 lights its engines, it's velocity is still about 310 meters per second. That's a little more than three times faster than Starship, before it lights it's engines for the landing burn. And it still hasn't even reached the equilibrium of terminal velocity. Because at this point it's still experiencing aerodynamic drag of almost two Gs So it didn't even reach terminal velocity period.
So I guess that's maybe a huge difference, is that Starship will actually hit terminal velocity and the Falcon 9 just doesn't. Okay, well, so what? 220 meters per second difference? That doesn't sound like that big of a deal. I mean, to get into low Earth orbit, you need to go about 7,800 meters per second. So 220 meters per second, that's only a small fraction of orbital velocity. Why is this belly flop maneuver worth it? Well, here's the problem.
When you're falling, every second you're trying to slow down propulsively, the first 9.8 meters per second of deceleration are just wasted fighting gravity. So that 235 meters per second can actually be a lot more because of something called gravity drag or gravity loss.
But in order to actually understand gravity losses, we need to first explain thrust to weight ratios and engine throttling. And here's where the fun begins. (bright music) Thrust to weight ratio. Perhaps you've heard of it, perhaps you've played lots of Kerbal space program like me and you have a pretty decent grasp of it.
Or maybe you don't have any idea what I'm talking about at all. And that's fine. So let's imagine a rocket hovering.
For now, let's completely ignore the atmosphere. If we separate these forces and concepts, it's going to make this a lot easier to learn. So in order to hover, the rocket engine needs to produce exactly as much thrust as the rocket weighs.
And in order to explain this best, we're going to use Newtons for both the weight and the thrust of the rocket, since it's a unit of force. An object with a mass of one kilogram weighs 9.8 Newtons on Earth. This is because Earth's gravity pulls at one kilogram with a force of 9.8 Newtons. And just for fun on Mars, the same mass would weigh 3.7 Newtons. Of course, it'd be just as easy to use pounds and pounds force in this example but we'll use Newtons despite me not being very used to it but it's all relative anyway.
So if your rocket weighs 1000 Newtons, otherwise known as they kiloNewton and you're producing 1000 Newtons of thrust in the opposite direction, you would hover because you have a thrust to weight ratio of 1:1, which means your thrust is exactly counteracting gravity and therefore your weight. So your net acceleration is zero because your thrust is exactly counteracting Earth's pole on your rocket. Produce 900 Newtons of thrust with your 1000N rocket and your thrust to weight ratio will be less than 1:1, specifically 0.9:1 and you'll go down. For each second you're at this throttle setting with that thrust to weight ratio of 0.9:1, you'll go downward faster and faster. You'd be accelerating downward.
And if you throttle back up to 1:1, you wouldn't go back to a hover magically. You'd actually continue to go down at the same velocity. A thrust to weight ratio of 1:1, just means your velocity is not changing.
So in order to get back to a hover, we need to increase our thrust to weight ratio to over 1:1, just to accelerate enough to reach zero velocity. So now let's throttle our engines to produce 1,100N of thrust, which would be a thrust to weight ratio of 1.1:1 and we'll start canceling out the velocity. Once we get back to zero velocity, we can return to a thrust to weight ratio of 1:1, if we want to hover. So now let's get back to where we started.
Let's go to thrust to weight ratio of 1.5:1 and accelerate quickly upwards. And again, this is very important to remember, if you instantly throttled back to a thrust to weight ratio of 1:1, you would continue going up at the same velocity. You wouldn't magically hover. So to get back to hovering where we started, we'll hold our upwards velocity until we are close to where we started. And then we'll reduce our thrust to weight ratio below 1:1, decelerate until our velocity is at zero meters per second and then increase our throttle back to a thrust to weight ratio of 1:1, to maintain a hover right back where we started.
It's actually quite hard to make a rocket hover and maneuver but it gets even more complicated when you remember that when a rocket engine is running, it's also burning fuel. So the rocket is getting lighter and lighter as propellant is expelled. So in order to maintain a thrust to weight ratio of 1:1, you have to be able to precisely throttle your engine to produce exactly as much thrust as your rocket weighs, even though it's getting lighter and lighter. And of course throttling an engine is a big deal for landing. Otherwise, if you couldn't throttle, you'd have to turn on your engines at the exact right moment.
Huh? That sounds like a really bad idea, right Joe Barnard? Okay, so let's take a look at Joe Barnard, from BPS spaces model rocket landing attempts, which he tried to do exactly this by using solid rocket motors, which can't throttle or shut down early. If you start your landing burn too early and have too much thrust and you hit zero velocity above the ground and the engine is still running, you're going to go back up. (rocket sound) If you start your landing burn too late, you're not going to be able to scrub off all your velocity and time and you'll smack into the ground. Or if you're like Joe Bernard's rockets, you're going to turn into a beautiful land shark.
Or here's another fun example. The Falcon 9 has too much thrust with only one of its nine Merlin engines at minimum throttle to be able to hover. So they have to start the landing burn very precisely.
This is called doing a hover slam or a suicide burn. If SpaceX started too early, they could run the risk of running out of fuel or hitting zero velocity before they touched the ground and would fall from even higher altitude. And of course if the Falcon 9 starts its engines too late, it will engage in an unscheduled disassembly, when it hits the ground at a very high velocity turning it into a Falcon million pieces.
Since a single Merlin engine can throttle between 100% down to about 40%, you can start your landing burn at a point where your throttle is directly in between the two, so about 70%. This allows you to increase your throttle, if you started a little late or decrease your throttle, if you started a little early. The rocket is continually calculating its velocity, it's deceleration and trying to make it hit zero meters per second, right when it hits zero meters in altitude. It will precisely control its throttle right up until touchdown. Although this is all done autonomously by the flight computer, which can precisely calculate exactly how much and how quickly to slow it down, it isn't quite as scary as you might think.
In fact, you may have done this a lot. Like maybe every single day. (bright music) A lot of us actually do a hover slam or a suicide burn, basically every day if you drive a car. So today we're actually going to be practicing our own hover slams and suicide burns and showing you that by modulating your brake pedal, you're basically doing the exact same thing as a rocket engine slowing down. So I'm going to be doing this with my friend Trevor Moreman.
So let's say you're traveling at 50 kilometers per hour and there's a Stop sign ahead. We want to try and stop right at the Stop sign without ever letting off the brakes and without ever touching the accelerator pedal again. And this example, the Stop sign is the ground, we're the rocket falling and we're using our brakes as the rocket engine that is slowing us down. But we can even go a little bit deeper with this example, since letting off the accelerator of your car is like lighting up your rocket engine. Because you begin to slow down before you even use your brakes. This is called engine braking.
And although it's pretty obvious in lower gears of an internal combustion engine car, it's even more obvious in a hybrid or an electric vehicle, which have regenerative braking. And with the Tesla, we can even change our regenerative breaking setting to be standard or low, which would be the same as changing the rocket engines' minimum throttle setting. So the next time you're driving around town, think about when you let off your accelerator, you just lit up your rocket engine, which will start slowing you down.
And as you use your brakes, it's like throttling your rocket engine more or less. It's pretty fun. Okay, so that's thrust to weight ratio and hover slams. So how has that affect this gravity drag/gravity loss thing? (bright music) Okay, so what the heck is this gravity drag or gravity loss thing? Gravity loss is when you're using your engine to fight gravity. So let's assume you have a thrust to weight ratio of 1:1.
As we know, you're not changing your velocity at all, your engines are just fighting gravity. So every second, you're running your engine with a thrust to weight ratio of 1:1, you're just wasting precious propellant fighting gravity. Your engine is trying to accelerate your craft at 9.8 meters per second squared and so is gravity, just in the opposite direction.
Now, like we talked about to actually get somewhere, we need a thrust to weight ratio of more than 1:1, since the first bit of propellant will always be sacrificed to that pesky gravity. And this makes a big difference in the overall efficiency and performance of a rocket. So let's plot this out because it's pretty fun to see spelled out.
On the left, we'll show thrust to weight ratio versus how much of the propellant is actually performing work, which is basically the efficiency of the burn against gravity. On the right, we'll show the thrust to weight ratio versus acceleration. So how much net acceleration the rocket is experiencing. If our thrust to weight ratio is 1:1, 100% of our propellant is spent fighting gravity, and 0% of our propellant is getting us somewhere, and as you know, that means our net acceleration is zero.
If our thrust to weight ratio is 1.1:1, 91% of our propellant is still spent fighting gravity and only 9% is used getting us somewhere, with the net acceleration of 0.1Gs. But this produces infinitely more work than a thrust to weight ratio of 1:1, since that wasn't producing any. So let's jump up to a thrust to weight ratio of 1.5:1. Now two thirds of our propellant is wasted to gravity and one third goes into accelerating the vehicle.
So although we only increased our thrust 36% over a thrust to weight ratio of 1.1:1, we actually produced five times the amount of net acceleration, getting us to 0.5Gs. Let's do it again now with a thrust to weight ratio of 2:1. Now 50% of our propellant is wasted on gravity and 50% of our propellant goes to accelerating our vehicle. Compared to 1.5:1, we only increased our thrust by 33% but we produced twice the amount of acceleration.
If we have a thrust to weight ratio of 3:1, only one third of our propellant was wasted fighting gravity and two thirds was used to perform work. Which is great, so we increase our thrust by 50% and we still doubled our acceleration. And lastly, if we have a thrust to weight ratio of 6:1, gravity only eats up about 17%.
So again, we doubled our thrust and more than doubled our acceleration. But notice we went from 66% of our propellant producing work at a thrust to weight ratio of 3:1, up to only 83% with a thrust to weight ratio of 6:1. So we're definitely seeing some diminishing returns. And no matter how high your thrust to weight ratio is, you'll never reach 100% of your propellant performing work because gravity will always eat 9.8 meters per second squared here on Earth. But percentage of how much of your thrust gravity is eating, just keeps going down until it's not really a major factor in the efficiency of your landing burn.
So let's think about a Falcon 9 falling from the sky again. If it's thrust to weight ratio is really low like 1.1:1, it would have to start its landing burn very high up and most of its propellant would be wasted just fighting gravity. It takes substantially more propellant to start your burn this high up.
Or think of it the other way. If you had a much higher thrust to weight ratio, it could do a very short landing burn, lighting it's engines at the last second and only a small fraction of the propellant was wasted fighting gravity. But the reality is it's actually not quite as bad as we just made it seem, at least here on Earth with Earth's atmosphere and especially towards the beginning of a Falcon 9's landing burn. Now this is really getting into the weeds on all this but when the Falcon 9 is near terminal velocity, drag alone is already producing an awful lot of deceleration.
There's much more drag than gravity is pulling down on the rocket, so it's slowing down before the engines even light. But once you light your engines, you of course begin to slow the rocket down but ironically, you're actually reducing the drag the rocket experiences. So the slower the rocket engine makes the rocket, the more work it actually has to do to fight off gravity. Near the end of the landing burn, it's basically carrying all the weight of the rocket and our whole thrust to weight ratio thing really matters here the most.
It's honestly crazy to me that engineers have to factor in all of this stuff, when they're designing these landing profiles. Okay, so now we know about terminal velocity. We know about gravity drag and how a high thrust to weight ratio can help combat it.
I think it's time we get into why does SpaceX do the flip from belly flop to tail down, so late? (bright music) So we finally have all the pieces of knowledge necessary to explain why they want to do the flip pretty much as late as possible. As you know, the terminal velocity of going belly first is much slower than engines down. So the longer they in that belly flop position, the less work the engines have to do. Cool, easy, but next we actually have a very carefully planned and wonderful compromise of mission planning.
Every decision has a compromise. So here's where things get pretty fun. So let's make an assumption.
We want to be belly flopping for as long as possible to scrub off velocity. But we also want to land safely and reliably, right? So we do want some wiggle room there and not be doing this last second landing burn, where there's absolutely no margin for error. What options are there? Starship has three C-level Raptor engines, all capable of aiding and the landing flip and landing burn. Each engine can throttle between about 40% and 100% throttle setting. So we can actually have some crossovers and thrust options, if we're running on one engine, two engines or three engines. With one engine running, we can produce between approximately 880 kilonewtons to 2200 kilonewtons of thrust.
With two engines running, we can produce between 1,760 kilonewtons to 4,400 kilonewtons of thrust. And with three engines running, we can produce 2,640 kilonewtons to 6,600 kilonewtons of thrust. And a good point of reference here, when Starship is falling from the sky before it lights its engines, it weighs approximately 1.4 million Newtons, otherwise known as 1.4 mega Newtons or 1400 kilonewtons.
That's about one mega Newton of weight for a dry Starship and about 400 kilonewtons worth of weight for the propellant that's leftover for landing, with a little bit left over for safety margins. Now, of course, these numbers are subject to change, as Starship gets more and more refined. It'll probably get a little bit heavier because it has more our heat shield but we could see the header tank shrink or whatever. But for now, let's just use this as a reference. We actually have a ton of engine options here but right away, you may notice.
we actually have a little bit of a paradox. If you want to start the flip early, you'll need a low thrust to weight ratio. So say one engine at a low throttle setting, which is the least reliable thing, as if you lose an engine, it may take too long to get the other engines up and running. But if you want to run all three engines the whole time, you'll have to start the flip scarily late and awfully close to the ground because with all three engines running at low throttle settings, it would still be a pretty aggressive suicide burn. But SpaceX has the option of lighting all three engines for the flip and then reducing the number of engines running, once the vehicle is closer to the ground, so they can have greater fine throttle control, while maintaining some redundancies at engineering mission, which seems like a decent compromise. But the performance hit of starting too early is actually quite a big deal.
In fact, I had Declan Murphy show the differences between the Delta V requirements for your landing burn at two different altitudes. And real quick reminder here of what Delta V is. It means change in velocity. And for rockets, you can kind of think of it like the range of a car. It's the balance of the fuel efficiency of your engine and how much fuel you have in your car.
So the higher the Delta V you have, the further you can go. So about the absolute earliest Starship can flip, is 2.5 kilometers. And that's flipping with two engines, then going down to one engine at nearly minimum throttle setting for the entire landing burn and using basically as much header tank fuel as possible. Then about the absolute latest Starship can flip, is down around 300 meters. And that requires all three Raptor engines running at their highest throttle setting, full bore, pulling 4.5 GS to come to a soft touchdown.
So now between these two options is some reasonable compromise. Likely right around the 550 meter mark, like we've seen SpaceX trying the flip so far and this balances some engine out capabilities and allows for some engine overlapping thrust profiles and it doesn't have a long and inefficient landing burn. Because when you compare their Delta V requirements at each of these altitudes, you can see there isn't a huge difference between 550 meters and 300 meters but there is a fairly substantial performance difference, if you start up at 2.5 kilometers. The result of that Delta V savings could be as much as almost 20 tons of propellent, which could mean slightly more than 20 tons that you could have put into orbit. Which is more than a single Falcon 9 has ever launched into orbit, period. So that's a lot of mass.
But of course it's important to remember for now, with its header tanks nearly full, it's around 30 tons of fuel anyway. So they might as well use all of it and do whatever is the safest. But maybe in the future, we could see the header tanks shrink as they aim to increase performance, while still having some redundant options for landing. But 2.5 kilometers is about the earliest
Starship can do the flip, period. Due to the size of the header tanks, which again of course, could always change but then you're just making it more inefficient and not really any more reliable, in any conceivable way if you were flipping earlier. 'Cause let's stop and think about it. So far of the four Starship prototypes that have flown as of the making of this video, none of them really would have worked out any better, had they started the flip earlier.
The exception being maybe SN 10, which had a problem where it was sucking up some of it's helium instead of propellant, which means the rocket hit the ground at too high of velocity. Now, of course there might always be some missions that have some unique edge cases or more extreme requirements. For instance, a heavier payload during landing, may always require all three engines running at nearly maximum throttle for landing. But that might not be a safe or reliable solution for humans.
To me, it seems like what they're doing now with lighting up three engines at the flip is great. Because that way, if one of them isn't working out okay, they can just continue to do the flip and the landing on two engines. Okay, so all of this will allow a Starship to fall slower and when timed correctly, it'll use very little propellant for the landing burn, which will increase the performance of the rocket, since the propellant saved can be used to put more stuff into orbit.
And that's the whole point. So the real goldmine for SpaceX will be finding that exact right altitude that balances performance, engine shutdown opportunities with overlapping thrust options between the engines and leaves them with the fewest chances to make a big old boom. But aren't big, huge booms inevitable no matter how reliable you make it? How is SpaceX actually going to make this reliable enough to make it safe for humans? Can they? (bright music) Okay, let's say everything works out perfectly and Starship can land reliably. This maneuver still looks pretty intense and isn't it always subject to some spectacular failures? What would it be like if humans were on board Starship, can it be safe? Is this something your life could depend on? As someone who wants to ride this thing now for dear moon, we better get into this.
Well, first off, the biggest weakness. We need to remind ourselves that for now, the Raptor engines are still in their infancy. They're literally still in development and they're getting more reliable by the day. They are far from perfect but SpaceX is flying and testing these things at a blistering speed and they're accelerating.
So with general engine reliability, the reliability of this maneuver will go up drastically, like we just talked about. By having landing profiles that will have few single point failures, it could become much more reliable. And on top of this SpaceX will practice this maneuver a ton, since they're planning on flying Starship in insane amount, between cargo and eventually refueling flights and all these other things, not only in this testing phase but once it's operational, it'll see an awful lot of flights and they'll have plenty of time to learn from successes, close calls and failures.
But there is something that will hopefully help make this flip maneuver itself a little bit more reliable, if there are problems with say the engine gambler or something, because SpaceX is working on powerful, hot gas thrusters, that use high pressure gaseous methane and high pressure gaseous oxygen, that can aid in the flip maneuver. But what if they didn't do that flip at all? Would the hot gas thrusters be powerful enough to just simply land belly first? Well, no, the hot gas thrusters will not be nearly powerful enough to be able to actually slow an entire Starship. But if you had enough of them, of course, you could maybe do that but lugging around all those additional engines and that extra plumbing, adds a lot of complexity, a lot more failure points and a lot of weight. Besides that, they'd have to beef up the rocket to handle landing it horizontally but more importantly, Starship already has Raptor engines, which are arguably some of the most incredible rocket engines ever made and will substantially outperform hot gas thrusters in every metric.
So why would they want to land on less efficient engines? But the same thing is basically true for parachutes. I have a lot of people asking me why they aren't using parachutes as a backup, if the main engines didn't light or something like that. Well, the first and most obvious reason for parachutes is that they take a long time to deploy.
And you have to deploy them in stages, so you don't create too much shock and destroy the chutes and or the lines. If Starship is lighting its engines up at say even 2000 meters and something goes wrong and they need to then deploy the parachutes, that's not even enough time to deploy the small droge shoots, let alone the main chutes. Not to mention the sheer weight of the parachutes and parachute system. They actually weigh quite a bit between the different shoots, the mortars that deploy them and of course, again, beefing up the structure, to handle the loads in that orientation and from the parachute mounting points.
And let's not forget about the sheer mass of Starship. At 100 metric tons when it's dry with absolutely zero fuel on board, it would be the heaviest thing to ever be carried under parachutes. The previous record was the space shuttle solid rocket boosters, which weighed about 90 tons. That's right, the space shuttle's SRBs were actually the largest parachutes ever. And they had three droge shoots and then there are three main shoots, which weighed around five tons just for the parachutes. Again, not to mention the mortars that would fire it or the additional structure for mounting the parachute to.
Okay, forget backups parachutes or backup engines and stuff like that. Let's say the flip just becomes 99.99% reliable for landing. Can a human even withstand the G-forces of the flip maneuver? Well, for that, let's take a look at the peak Gs of the landing maneuver. Believe it or not, the peak Gs of the flip and landing is under three GS. Now, granted they may be in a slightly dizzying mixture of vertical and horizontal, but they're not even as great as many rollercoasters.
And of course there likely could be seats that would rotate and make it so you always experienced G-forces in the same direction, mostly lying on your back to spread out the GS as much as possible, to keep you safe. And just look at the actual maneuver. Follow the nose where the passengers or cargo would be someday.
It doesn't actually move that much at all. Yes, the tail whips around pretty aggressively but the nose barely does at all. I think it just looks scarier than it is. At the end of the day, I'd rather experience the Gs of Starship than the Gs of a Falcon 9 booster propulsive landing, which pulls up to five Gs at certain points during re-entry and landing burns.
While that's certainly survivable, it's definitely starting to get pretty uncomfortable. Now of course, no one's riding a Falcon 9 booster when it lands, but it just shows how high the G-forces are, in order to recover a booster vertically. So although the belly flop looks dangerous and nauseating, it likely won't actually be that bad for humans. Especially if the seats compensated for the rotation, it'd probably be no big deal at all.
But as far as reliability, well only time will tell how reliable this actually can be. So with that, I definitely think we need to wrap things up. (bright music) So SpaceX is trying something pretty spectacular.
And there's reasons to maybe be skeptical about it at this point, having seen so many failures but let's not forget this is just the very beginning. The Raptors aren't very mature yet either and we'll definitely get more reliable, as they continue development. For those of you that weren't around during the early days of the Falcon 9, many people had their doubts that SpaceX could even get it to fly, ever. There were scrubs galore, problems and questions coming up every time they got a Falcon 9 to the pad. And then of course there many doubts, if they could ever get it to land. And at first it did seem like a bad idea and like something that just might never be worth their time.
But now, no one doubts the merits of SpaceX landing and reusing their Falcon 9 boosters. As of today about 75% of all Falcon 9 flights have successfully landed and been recovered. And about half of all missions have been on reef flown boosters. They clearly have figured out a system that works incredibly well and has already changed the game.
Or perhaps you remember when SpaceX started using super chill propellant for their Falcon 9. They were scrubbing constantly. For instance, SES Nine, one of the first missions to use super chill props, was scrubbed four times because of issues with the super chilled propellant. And people were questioning, whether or not it was worth it and if they could ever get it to actually just work out without being super finicky, including ULA's, Tory Bruno. But the ultimate question, why? We've said it at the beginning and we'll say it again now that you understand everything.
Using as much of the rocket to slow down in the atmosphere is possible, essentially for free, that's a great reason right there. And then by doing the flip as late as possible, they minimize gravity drag and they won't waste too much Delta V landing the rocket. In order to fulfill the goal of being a super heavy lift, fully reusable rocket, they have to squeeze absolutely every little drop of performance out of the vehicle. And the early days of this testing program, we're going to see a lot of failures.
SpaceX will learn a lot of lessons but they're cranking these things out so fast, they can rapidly iterate and learn at a pace that will certainly lead to getting results in a hurry. But the reality is, I know the last minute aspect looks scary, but frankly every part just simply needs to work. This all just needs to become more reliable period. And if I know anything about SpaceX, they will force this thing into being reliable because they don't seem to take no for an answer.
After all nothing that's physically possible is impossible. But at the end of the day, who knows, SpaceX could play around with this for a while and then say, "You know what? Let's just focus on what gets us the absolute highest chances of safely landing and recovering period." And then they could make some just drastic changes. I mean, Elon's already talking about catching the booster with the launch tower and maybe even doing that same thing with Starship.
Who knows, maybe someday we'll see them blowing a bunch of fans up at Starship and catching it in a giant net. I mean, I feel like nothing is off the table for SpaceX, but for now I'm excited to see them try this more because it sure is exciting. So what do you think, do you think SpaceX will figure out this maneuver and make it reliable and routine? Or do you think they'll wind up back at the drawing board and have to come up with another completely different solution altogether? Let me know your thoughts in the comments below. I owe a quick thank you to a lot of people who helped make this video possible, including Declan Murphy from flightclub.io. You guys should definitely be familiar with this, if you're out ever trying to take pictures of a rocket, be sure and check out his rocket photographer toolkit. There's a ton of really fun stuff to play with though, too.
So definitely check out flightclub.io. It is awesome and Declan does amazing work and check him out on YouTube too, cause he's doing an awesome job explaining some of the differences we're seeing between these missions. And also Casper Stanley, who made a lot of the awesome 3D renders in this video, check out his rocket Explorer app on the steam store, which is super cool. And another thanks to Corey @C_bass3d on Twitter, for some of his incredible animations as well. And a lot of the footage you saw from this video was from the partnership I have with my friends at Cosmic Perspective.
So Ryan Shilanski and Mary Liz Bender, who worked with me to make a lot of the incredible video that you see on launch day. Definitely watch their incredible films on YouTube, find their YouTube page right now. And once you watch their videos, I can just about guarantee you're going to want to support them. So you get some awesome access to behind the scenes stuff and help them continue to do what they do, by going to patreon.com/cosmicperspective. But I owe a huge thank you to my Patreon supporters, who helped make this video, Mars studio B and everything that we do at Everyday Astronaut possible. So if you want to help support what I do or gain access to our incredible discord community, who is just the most fun audience to be with on launch day or just hang out during the week, asking people rocket questions and stuff like that.
Or if you want to get access to some exclusive live streams as well, consider becoming a Patreon supporter by going to patreon.com/everydayastronaut. And while you're online, definitely check out our awesome web store for shirts like this shirt, that I'm sure you've seen many times. This is the full flow stage combustion cycle shirt, that of course you've probably seen Elon Musk wear or his baby or whoever elsewhere, it's an awesome shirt. All of our merch is the highest quality possible by screen printing them. And we just do bulk orders of merchandise, it's not print on demand stuff that will fall apart after three times wearing it. So definitely check out, shop around, find some awesome stuff for you or some friends, by going to everyday astronaut.com/shop.
Thanks everybody. That's going to do it for me. I'm Tim Dodd, the everyday astronaut, bringing space down to Earth, for everyday people. (bright music)
2021-04-24 21:10