Heat Pumps: the Future of Home Heating
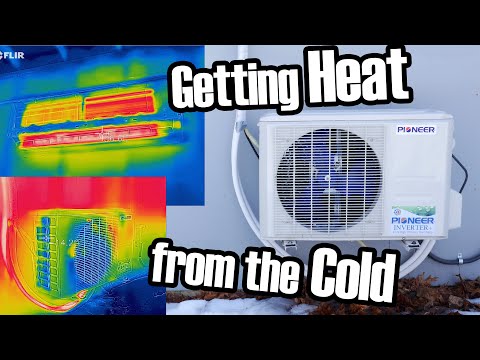
Well, I’m pumped. What if I were to tell you that all of the energy you need to heat your home on a cold winter day could be found… outside? And that if you could capture the heat energy out there and move it inside your home, you could make yourself comfortable using a fraction of the energy of other methods? Sounds impossible at first, doesn’t it? If it’s colder outside than in, what heat is there to take? But it’s very possible, and thanks to heat pumps we can do it right now. And that’s what this video is all about. Heat pumps are simultaneously a very old yet also emerging home heating technology. Using a heat pump under ideal conditions requires as little as one fifth the energy of ordinary electric heat.
With a coefficient of performance of 2.5 or greater, a heat pump will produce more heat for you per unit of fuel burned in a power plant than you could get by burning that fuel onsite in your home. This tremendous efficiency all but guarantees that the heat pump is the heating technology of the future. But what is a heat pump? Ah, well here’s where we need to talk about some terminology and also who the primary audience of this video is. I’m making this video mostly for an American audience because we’ve gone about this in a weird order. We’ve had air conditioning in a lot of homes (and cars, for that matter) for a very long time now.
Air conditioning has been more or less standard equipment in American homes for more than 50 years, even in a climate like mine where winters can be brutally cold. And in places like the Southern US, air conditioning is practically a requirement for basic survival. And the thing is, air conditioners ARE heat pumps. Yet heat pumps, especially in regions like mine, are a rather new thing.
Confused yet? Well, in some other parts of the world what we call a heat pump is referred to as a reverse cycle air conditioner, and that should give you a pretty solid idea of what’s going on. But, because this wouldn’t be Technology Connections without an explanation of the refrigeration cycle, let’s get down and dirty in the nitty gritty of latent heat and the magic of refrigerants. If you’d like to skip this part, skip to [a computer voice reads 7:38] Let’s start with a refrigerator - nearly everyone has one of those. What does your refrigerator or freezer do exactly? Well, it needs to make its insides colder than its outsides.
That’s trickier to accomplish than it seems at first glance. It has to get heat energy out of itself. See, that’s the thing about temperature.
What we call temperature is really the concentration of heat energy in a given space. If there’s a lot of energy crammed in a space, it’s hot. If the energy is really spread out, it’s cold. And thanks to entropy, energy always wants to spread out, or move from areas of high concentration to low concentration. This freezer’s insides are at about 5 degrees below zero. But the room it’s sitting in is much warmer than that, about 65 degrees.
Because the energy around the freezer is more concentrated, ambient energy wants to spill into it. Wherever there’s a temperature gradient, nature is hot and bothered, frankly, and would very much like it to achieve equilibrium. So eventually the insides of the freezer will warm up.
We can slow that down by adding a whole bunch of insulation or tweaking its design (in fact, this freezer and this footage was featured in a video discussing that very topic) but eventually we need to work to reverse this process and keep its insides cold relative to its outsides. And to do that, we use a heat pump. A refrigeration system collects heat energy in one place and disperses it in another. It pumps heat.
We’ll get into how it does that shortly but the key thing I want you to recognize here is that even though the inside is very cold, there is heat energy in there. The freezer’s heat pump is collecting it from a very cold place, yet in doing so it creates warmth. The sides of the freezer get warm when it’s running, and that’s the heat it pulled from inside itself being rejected into the surrounding air. What makes this possible is a refrigerant. Refrigerants are gaseous chemical compounds with a particularly useful property: easy to manipulate boiling points.
For example, R-134a boils at 15 below zero, or -26 Celsius. That’s quite cold. But here’s a can of it. It’s a liquid in here. How can that be? Well, like any chemical, its boiling point is affected by ambient pressure.
You know how the boiling point of water is defined at sea level? That’s because higher up in the atmosphere where there’s less atmospheric pressure, it’s easier for water to boil. A rather crude way to think about it is that being under pressure squeezes all the molecules together, and that force provides an additional barrier to changing phases from liquid to gas. When there’s less pressure, it can change phases a bit more easily which lowers the amount of heat energy it needs to start boiling and thus its boiling point.
When there’s more pressure, everything is held tightly together and the opposite occurs; its boiling point goes up. Because this is trapped in this can, it's under higher pressure and can remain a liquid. But when anything changes phases, it needs to absorb or release heat as it does so. This latent heat isn’t something we can feel directly, but it is a tremendous amount of energy. For example, getting a given quantity of water to vaporize takes about seven times as much energy as it does to bring it from room temperature to its boiling point. In other words, if it took 10 minutes to bring a pot of water to boil on high heat, it will take 70 minutes on top of that for it to all boil away.
That extra energy is being used to free the molecules from their liquid phase into the vapor phase, but it doesn’t make the water any hotter. This is, incidentally, the principle on which automatic rice cookers work. Another plug? Why yes! How ‘bout a third one? We just saw what the release of latent heat is like in the reusable hand warmer video. Simply by falling from the liquid phase into the solid, the sodium acetate gets hot. Its latent heat of fusion is being released as it crystalizes, which means it gets warm - about as warm as its melting point. To reset it, you put a solid hand warmer in boiling water where, now that it’s colder than its surroundings, heat will spill into it, causing it to melt.
As it does so, it re-absorbs its latent heat of fusion, and thanks to the fact that it can be supercooled without freezing it can store it for later use. Refrigerants, just like water and sodium acetate, have to absorb and release latent heat to change phases. What makes them useful is that thanks to their flexible boiling points, we can force this to happen and use it to our advantage. All we need to do is create a machine which allows us to manipulate the ambient pressure the refrigerant experiences. Refrigeration systems are nothing more than a closed loop of piping filled with a refrigerant that exploits its latent heat of vaporization to move energy.
Using a compressor to create a pressure gradient allows us to force the refrigerant to condense thus releasing its latent heat of vaporization. We can then force it to evaporate and reabsorb its latent heat. Do this in two different locations and you pump heat across a thermal barrier. Now I’d like to show you what this looks like. Those of you that skipped to here, welcome back! We’re still talking about how refrigeration works but I wanted you back for this.
Thanks to a student at Front Range Community College's Center for Integrated Manufacturing who had hands-on learning with these I have some great footage to share of a refrigeration demo rig. This is used to demonstrate all sorts of refrigeration technologies and learn how to troubleshoot them, but for now I just want to highlight the basics. Inside these pipes is a refrigerant. If you follow them, you’ll see that they form a circuit.
The compressor is the heart of any refrigeration system (it does pump, after all) and it’s right here. Hermetic refers to the fact that the black canister hermetically seals an envelope for the mechanical compressor and its motor to sit inside without letting the refrigerant escape. On the left and right are two heat exchangers. These are the evaporator and the condenser.
They are also the condenser and the evaporator. See, they’re labeled both. Because they are. This rig’s operation is reversible, meaning whether the heat exchanger is an evaporator or a condenser is arbitrary. We’ll see how that works in practice shortly but what’s really neat about this rig is that we can see liquid refrigerant traveling through it. Many refrigeration systems will have a sight glass like this which is useful for diagnostics, but glass piping? That’s a rarity.
The refrigerant is only able to liquify because the pressure in this pipe is fairly high. It first entered the compressor as a low pressure gas, but being squeezed into the high pressure side raised its boiling point well above the ambient temperature. The act of compression also made it quite hot. The job of the condenser is to cool it back down, and it does this by forcing air through densely packed metal fins attached to the copper piping. This forms a heat exchanger with a large surface area to help transfer heat in the refrigerant to the air.
At this pressure, if the refrigerant were R134a, its boiling point would be raised to about 130°F, much higher than the ambient air temperature. After exiting the compressor it’s hotter than even that, but airflow through the heat exchanger helps cool it down. Once the refrigerant has cooled to its artificially raised boiling point, it will start to condense.
And as it does that, it releases its latent heat of vaporization. Now even more energy is being released from the refrigerant, and it will stay near its boiling point until the gas has condensed into a liquid. The upshot is that the condenser gets very hot. But where did that heat energy come from? Well, some of it came from the act of compression itself; simply compressing a gas makes it hot because you’ve squeezed its thermal energy into a smaller space, but most of it came from the refrigerant itself mere moments ago. See, after it’s all a liquid it gets held back by some sort of metering device at the end of the condenser. There are various types of metering device out there, the simplest of which is a capillary tube, and their job is to create a restriction in the refrigerant flow and thus maintain the pressure gradient we rely on to make this all work.
Once the liquid refrigerant makes it through the metering device, it finds itself in another heat exchanger, just like the condenser. But here, the pressure is very low thanks to the suction on the input side of the compressor. What exactly the pressure is will depend on the refrigerants used and also conditions, but the pressure is low enough that the boiling point of the refrigerant will plummet to below ambient temperature. For sake of explanation we’ll assume it’s zero degrees. And here’s the key: to boil, the refrigerant has to absorb its latent heat of vaporization. And now that its boiling point is below ambient temperature, it will spontaneously start boiling and get very cold.
To boil, it initially gets the latent heat it needs from itself which brings the remaining liquid down to the new boiling point. From there, it will pull energy from ambient air. See, now that it’s colder than ambient air, energy from the air will flow into the refrigerant. Remember, energy always wants to spread out, and now there’s a place that’s colder than its surrounding for that to happen. Thus the refrigerant is absorbing the energy from its surroundings as it boils. This heat exchanger is called the evaporator because that’s what the refrigerant does.
It evaporates here, and condenses over there. So, finally, here’s where we get into how we make this work for us and why heat pumps are so cool. I mean hot.
Hotly cool, or cooly hot. And why many American audiences may find this whole idea a little perplexing. See, a lot of us are used to energy flow in a refrigeration system going one way. We have refrigerators which make their insides colder. And we have air conditioners which make our living spaces colder. Refrigeration is for cold! See, here’s an air conditioner.
This is the condenser, that’s the evaporator. The evaporator goes inside. Condenser goes outside (unless of course you have a portable air conditioner). But, but but the job of the air conditioner is to take heat out.
Duh. Here’s a central air conditioner. This is the condensing unit. It has the compressor and the condenser. Heat comes out of here, and that’s - that's just the way it is. The evaporator sits above my furnace and makes the air colder and seven months out of the year, uh, sits pretty.
Here’s a mini-split air conditioner. This is the condensing unit, and inside is a self-contained evaporator and blower. Ah but this is also the evaporating unit, and inside is a self-contained condenser and blower! This is reversible, and so is also a heat pump. This mini-split heat pump system has a different form factor from the central air conditioning system next to it, but mechanically they're something like 95% identical.
There are some technological improvements in the mini-split like a variable-speed compressor and more intelligent controls which help it gain efficiency, but fundamentally the heat pump is no different from the air conditioner. It’s a compressor, a metering device, a refrigerant lineset, two heat exchangers, and a fan of some sort to force air through each heat one. The main difference is that the central system uses the furnace as its indoor blower motor, and the mini-split has one of its own in the wall-mounted unit.
But the minisplit has one additional component that changes it from a cooling-only device to efficient year-round climate control. Let’s start with a closer look at it. This is a 1.5 ton unit, or 18,000 BTU or about 5250 watts. The outside unit contains most of its guts and unfortunately is not very easy to take apart. That’s not that important, though, as we can see the heat exchanger and refrigerant hoses. They’re here.
And here. The fan forces air through the heat exchanger which wraps around the side so we can see its piping and fins. The inside unit is practically the same thing but it doesn’t have the compressor and the fan’s a linear blower type thing. Lift this up and there’s the other heat exchanger, plain as day.
The fan forces air through it and exhausts through this swinging vent guide thingy. The metering device also lives here, I believe it’s a simple capillary tube - uh, please correct me if I’m wrong. In any case, it lives at the end of the liquid line, that’s the smaller of the two, and is the very last thing the refrigerant goes through before it enters this heat exchanger. Sometimes.
Let’s get out the thermal camera and observe this thing. Note that the displayed temperature is likely inaccurate due to different emissivities of materials, but it’s the visuals that are more important anyway. When it’s not running, there is no pressure gradient and the refrigerant pressures in both heat exchangers are roughly equal. In cooling mode, the compressor works to pull gas out of this heat exchanger and lower the pressure inside of it.
This lowers the boiling point of the refrigerant, and any liquid refrigerant remaining in the heat exchanger boils away. This causes the heat exchanger to get very cold - in this mode, it’s the evaporator and the refrigerant absorbs latent heat to boil. It has become a thermal sink and energy in the room is naturally moving into it, sped up with the help of the fan. That ultimately makes the air colder. Meanwhile the outside unit is getting warm. The compressor is forcing the gas it just pulled out of the evaporator into the condenser.
Under this higher pressure, its boiling shoots up - way above the ambient temperature out here, so the refrigerant will condense inside the pipes. That makes this the condenser, and the very energy that just got absorbed inside as the refrigerant boiled is now being expelled out here as it recondenses. That heat energy was pumped from inside to outside, and that’s what air conditioners do.
But here’s where things get interesting. Let’s ask a simple question. What if the roles could be reversed? Is it possible to collect heat energy in the outside unit and expel it from the inside unit? That would be pumping heat but in the other direction. Well, of course, it is absolutely possible and frankly stupidly easy to accomplish.
All you need to do with a basic system like this is move the refrigerant through it backwards. That’s it. Now, you can’t just run the compressor backwards.
Refrigerant can only go through it in one direction. But with just a little bit of extra piping and a special valve, you can change the direction it flows through the rest of the system. Virtually the only difference between this mini-split unit and the central air conditioner next to it (aside from the variable speed compressor and a few other particulars) is that the mini-split has a reversing valve and its associated piping. This allows refrigerant to flow in the opposite direction, and that flips the system on its head. Remember how in cooling mode the restriction was just before the evaporator? High-pressure liquid refrigerant built up right before it entered this space at the capillary tube. It would then evaporate in this coil once it made it through because the pressure in here was quite low.
But, if refrigerant is going the other way, well now the restriction is at the end of this coil! High-pressure liquid refrigerant will build up inside of here, turning this into the condenser where the refrigerant will reject latent heat. Once it makes it through, it finds itself on its way back outside in a low-pressure environment where it will boil and absorb latent heat from the air. That makes the outside unit the evaporator.
Take a look. Here, the mini-split is operating in cooling mode. The inside unit is the evaporator, absorbing heat, and the outside unit is the condenser, rejecting it.
This is how we expect air conditioning to work. But switch it into heating mode and after a few minutes of idle time to allow the pressures to equalize, the reversing valve switches the direction of the refrigerant flow and the roles become reversed. When the compressor starts up again, the outside unit becomes the evaporator, absorbing heat in the refrigerant, and the inside unit becomes the condenser, rejecting that heat and warming the space.
In a sense, it’s air conditioning the outside air and using the heat collected from that process to warm the space. Here’s why this is a big deal. The actual work being done here is compressing a gas. Yeah a little energy is being used by the fans but nearly all the electrical energy this unit consumes goes into the electric motor which drives the compressor. The refrigerant changes phases in a natural, spontaneous process. We just create the right conditions for that to occur.
Because of this, this heat pump is able to move up to five and a half times as much heat energy as it actually consumes in electricity. That’s frankly amazing. It’s like running five space heaters for the cost of one.
And why does it work even when it’s cold outside? Well, remember that heat energy always wants to spread out. And also remember that the boiling point of refrigerants is very, very cold at low pressures. So long as you can get the evaporator colder than the air around it, it will be able to capture heat energy as the refrigerant boils. Here the ambient temperature was about 40 degrees, but the evaporator coil is much colder than that so it becomes a place ambient energy wants to go.
Once the refrigerant is compressed and brought to the inside unit, it will condense and release that acquired energy which warms the space. It doesn’t matter that the outside air has less energy in it than inside. All we’re doing when we heat or cool a room is affect energy concentration in that room. The outside air may have a lower energy concentration, but the refrigerant’s energy concentration can be made even lower than that. That’s all it takes - make a region colder than another and heat energy will always flow into the colder one.
Since the refrigerant will hold onto that energy and release it when it condenses, just compress it to raise its boiling point, move it inside and boom! Heat from the cold. But it's not all sunshine and rainbows. The trickiest thing about heat pumps like this (this is known as an air-source heat pump) is that as it gets colder outside, their effectiveness is reduced. The Coefficient of Performance, or COP, describes how much heat energy the heat pump moves compared to how much it consumes.
A COP of 1 is… bad. That’s just a one to one ratio, the same as resistive heating. But a COP of 4 is easily attainable under decent conditions. That’s pretty much any time it’s a few degrees above freezing. Once you’re close to freezing, though, well there’s a complication. You probably know that an air conditioner removes moisture from inside your home.
This happens because the evaporator’s cold surface causes water in the air to condense on it. Well, if the evaporator is outside... then it’s probably gonna collect some moisture.
It does. Now, that actually helps with heating since water releases latent heat when it condenses, but if it’s close to or below freezing outside… well that water turns to ice once the heat pump has gobbled up its heat energy. Over time, heat pumps like this build up a coating of frost on the outside unit. How quickly this happens depends a lot on environmental conditions - if it’s a dry day, it’ll happen slowly.
But if it’s humid, it happens pretty fast. And this reduces its effectiveness. Not only does ice on the coils provide a layer of insulation which slows the process of scavenging heat from the air, but eventually the ice impedes the airflow through the coil entirely by filling the gaps between the fins. How do we deal with this? Well, it’s actually pretty easy.
You can defrost the coil simply by briefly reversing the refrigerant flow. That will melt the frost that’s built up at the expense of pulling a little energy back outside. Since this unit has no other means of defrosting, it has to do it fairly cleverly. Sensors help it determine when it needs to defrost - a huge benefit of the unit being self contained like this is that it knows both the inside and outside temperature, and by monitoring refrigerant pressure it can determine how well it’s working. Observe here that prior to defrosting, it kept increasing the speed of the compressor to counteract the slowing effects of the ice.
[a mechanical buzzing which successively increases in pitch and intensity] Eventually, though, it decides to defrost. [buzzing stops] When it defrosts, it first stops the compressor and then stops both fans. That makes sure it doesn’t blow cold air inside and allows the outside coil to get hotter.
It then restarts the compressor in cooling mode, once again turning the outside coil into the condenser. The heat it’s pulling from inside quickly melts the ice. [compressor buzzing again] Once it’s concluded that it’s done, it stops, reverses the refrigerant flow again and starts the outdoor fan and compressor to begin collecting heat once more. But it waits to turn the indoor blower back on until the inside coil has warmed up a bit. To the user, a defrost simply seems like a pause in heating, but as you’ve seen it’s actually much more complicated than that.
However, this reduces its efficiency and ultimately its effectiveness. On cold, humid days it has to defrost rather frequently, limiting its output, and reducing efficiency. How do we measure efficiency? Well, because this is the US, we use weird units like SEER and HSPF. This unit has a SEER of 19, which is pretty great actually! That’s equivalent to a COP of 5.5.
But the seasonal energy efficiency ratio describes cooling. Its HSPF, heating seasonal performance factor, is 10. Luckily we can convert that to the COP, and that means this thing’s average coefficient of performance for heating is, at least according to how it’s tested, 2.9.
So over a typical heating season, you can expect this to deliver almost 3 times as much heat energy as it cost to run. Some days it’ll be better, others worse. Over time, though, it would need roughly a third the electricity of a plain resistive heater to produce the same amount of heat. But there’s a catch.
This thing is only rated to work down to 5 degrees. Now, it will work below that temperature! In fact, here’s some footage I made when it was ten below zero. [camera audio] Oh my goodness it's cold out here. Like it's, it's dangerously cold out here especially to be standing in front of it blowing colder than ambient air at me! Yeah, so I'm gonna go inside.
I know this is a terrible tool for this job but it’s what I had. It was still running and producing some heat, but to be fair it was pretty tepid. It was also running its guts out so its coefficient of performance in this scenario may have been just 1, or maybe slightly better. This particular heat pump just isn’t equipped to handle weather this cold. Which, to be clear, is fine for me.
This thing is in my garage and the heating is mostly a bonus. I installed it mainly to remove moisture because summers here are stupid humid. Seriously, I left the leather seats from my family’s old minivan’s in here my first summer and by the fall they were completely covered in mold.
Some other stuff got damaged, too, so if I wanted to keep anything in here of value air conditioning was practically a must. But it certainly is handy on milder winter days to be able to use this as a workspace. When it’s above freezing, the air this thing blows is proper hot! Like, it genuinely surprised me. In retrospect it shouldn’t have, 18,000 BTU is more than three US-spec space heaters. But knowing that the heat it produces is coming from outside makes that feel almost like magic.
And even when it’s colder and not that efficient, it has a freeze protection mode that keeps the temperature at 45 degrees, which is enough to melt the snow off my car after a drive and, well, that is a very nice luxury, I'll tell ya that. I usually leave it in that mode. However, it’s true that on its own this could not reliably provide me with sufficient heat throughout the winter. I would need a backup source of heat when conditions weren’t ideal for a heat pump.
That’s often electric resistive heating which will always work but needs a ton of energy to do so. But it could also be natural gas or another fuel. In whole-home systems with heat pumps, the backup heat is often called emergency heat or auxiliary heat, and many thermostats are designed to control these systems as one.
But, even in my climate, on many days a heat pump would be all I need. In fact, that’s the case for the vast majority of the winter. It’s not actually all that often that it dips below 5 degrees here. And still, some heat pumps like this are rated down to 20 below zero! It’s extremely rare for it to get colder than that here.
Heat pumps can be made to work in colder temperatures through different refrigerants, altered designs, or through electric defrost coils where the outside unit has dedicated heaters to allow it to defrost while still operating in heating mode. But let’s set aside those potential fixes. Here’s a question I’ve been asking a lot lately: Why isn’t this thing also a heat pump? This HVAC system isn’t even two years old but no one even bothered giving me the option for a heat pump. Yes, it’s cold enough where I live that a gas-fired furnace is the norm, but I’ve got 95% of a reversible heat pump sitting right here! This 2.5 ton air conditioner may only be able to put out half of what my furnace can with its fire tubes,
but on mild days - so the ones a heat pump would be best at - that’s more than I need anyway. Heck, even when it was -10 outside, my 70,000 BTU furnace only ran for about 10 hours a day. 30,000 BTU continuously would have covered that, but yes I know that output wouldn’t have been possible at that ambient temp.
I'm just spitballin’ here. And it’s not like heat pumps systems are unheard of in my neck of the woods. In fact, a patron of the channel has one and they’re actually a little farther North than I am! This outdoor unit is practically the exact same thing as the condensing unit for my A/C system, save for the reversing valve and a more complicated compressor setup.
It functions exactly as the mini-split does, doing the same periodic reversing to defrost. I mean, there’s snow on the ground yet it’s still working! Since this is almost the exact same machine as mine, it annoys me endlessly that reversibility isn’t just standard at this point. In fact, in the South reversible heat pumps in this form factor are pretty common, and have been for years. Since heating demand is usually mild if any in the South, relatively few folks have natural gas for heating.
When you’re all-electric and have air conditioning, you might as well have a heat pump to save money on heating costs. And since all it really takes is a reversing valve and a few other particulars to make that happen, I see it as a no-brainer. Well, usually. When a large, disruptive weather event takes hold that causes an entire region to suddenly need resistive heat because it’s too cold for their heat pumps to work effectively, that can put an untenable strain on the electric grid. When this video was published, Texas had just gone through one of these extreme events. Heat pumps certainly were not the cause of the grid failures, to be clear, as a lot of issues occurred on the generation side of things and based on what I’ve read, natural gas backup heat is more common than I thought.
But the occasional need for an energy-intensive backup heat source is good to keep in mind. But so far, we’ve just been talking about air source heat pumps. These are definitely the most common because, well, they’re easy and simple to make. But there are other sources of heat to be tapped into. And heat pump technology is finding itself in more and more places as we discover the benefits of moving heat rather than creating it.
In part 2, we’ll look at some of these solutions and discuss where heat pumps have to go from here. We’ll also look at some cost/benefit comparisons both from a financial perspective and from a climate change perspective. And that’s a key reason heat pumps will undoubtedly see more widespread use as time goes on. I said earlier that with a COP of 2.5 you’ll get more heating out of a heat pump
than you would burning natural gas onsite. Let me explain that. In 2019 the average efficiency of a natural gas power plant in the US was 44 percent according to the Energy Information Administration. Now, if you run electric resistive heat from this source of energy, after transmission losses you’ll end up getting about 40% of the energy out of the natural gas. Not bad, but you can also burn natural gas in an onsite furnace and get more than 90% of the energy out of it. It’s for that reason that areas like mine tend to have natural gas infrastructure capable of delivering it directly to homes.
Historically it’s been cheaper, easier, and more efficient. But, when a heat pump is running at a COP of 2.5, sure you’re still only getting 40% of the energy from the natural gas, but with it you’re moving 2.5 times that amount. That means that in the end, you’re getting the equivalent of 100% out of the natural gas.
And you can even get more than that! A heat pump is frankly the most effective way to turn electricity into heat, so even on today’s fossil-fired power grid, they’re a wiser use of limited resources and can help lower emissions. And they also make renewable energy sources much more feasible in cold climates. While you certainly can use the output from wind or solar to heat your home resistively, that takes a lot of output and makes storage hard to manage. If everyone had very efficient heat pumps, that energy demand could be cut into a quarter or perhaps a little less.
And while heat pumps like this struggle in cold weather, there is an alternative. The ground-source or geothermal heat pump is a way to attain year-round, near-constant peak efficiency with heat pumps even in cold climates. And we’ll talk about those, some other novel uses for heat pumps, as well as the need for more climate-friendly refrigerants in the next video.
For now, stay warm! ♫ coefficient of smooth jazz ♫ and that, if you could capture the heat energy out of... [goofy noise] well we're gonna restart this line because. Oh cripe. Eurggh! Just my terrible teleprompter causing me problems again. And the thing is, air condish... and the..pff heh.
Ehehhe. ...allows us to manipulate the ambient pressure the refrigerants experience. The S is on the wrong word. That line needs an on-the-fly re-write! Those are always great. ...boiling point shoots way up. Wayyu... the, there are words missing! This is, incidentally, the principle on which automatic rice cookers work.
Another plug? Dammit! I hope you're pumped for part 2. I know I am. Gonna talk about them coolant pipes. Digging wells. All that.
2021-03-01 14:54