Energy Solutions Forum: Christopher W. Jones
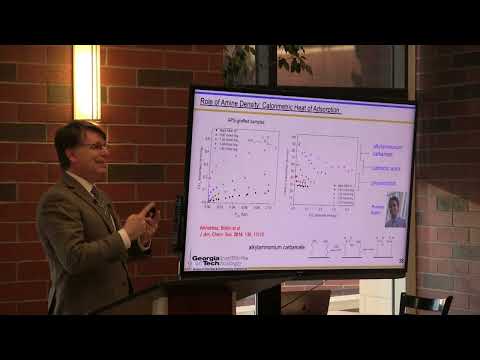
I'm really happy to have the opportunity to come and talk to you a little bit about the research that we've got going on at Georgia Tech, both in my group as well as in other groups. I'm going to try to keep the talk mostly high level, but for those who are chemists or chemical engineers in the audience, I'm going to also try to have about ten or 15 minutes of some hardcore science and engineering for you as well. So I'm going to talk about direct air capture, which I'll refer to as DC in this talk, which I believe is going to be chemical engineering's contribution to a grand societal challenge that all of you have mobilized to to hear about and to work around. Let's see if I can get my remote to work.
All right. So I'm going to start by introducing you to where where I live and work, which is in Atlanta, Georgia. It's a short plane ride away if you want to come and visit. But if you do take that plane, you should recognize that's going to be the largest carbon footprint activity that you're going to do probably.
And in that month, I'm fortunate to work at Georgia Tech. Georgia Tech's got a very strong college of engineering both at the graduate and undergraduate level. Many of the people in this room are mentors to young people who are interested in STEM, or some of you may be students or postdocs here as well. When you're done innovating here at in Reno, we would be very happy to see you in Atlanta.
My particular program in chemical engineering is one of the largest chemical engineering programs in the country, has about 40 faculty, 200 Ph.D. students, and about 800 undergraduates. It's going to take me a moment to figure out how to get this to work. 2.0 over here.
Okay. All right. All right. My finger is now working.
All right. So I'm going to start with a big picture message. And it's a message that I hope you will see as a message of hope. And that message is that we have solved big problems in the past, problems that are the same scale as the climate change problem.
Unfortunately, I would argue that the climate change problem that our generation of scientists and engineers are now tasked with tackling is a direct consequence of this previous problem that was solved. And so if we. Yeah, I'm going to need someone to help me with this.
It's not working. We can't see. I can. I can sit over there.
I also can give you another remote if you want. But let's just make it too far. Okay. I know what to do now. I'm going to come closer.
So this is good. It allowed me to move. So if we. If we take a step back in time to 1900 and we imagine a similar gathering like we we are here today talking about the grand challenge of the time, you know, what would that challenge have been? What would we have read about in the front page of The New York Times or or heard in mass media? And it would have been famine. So at the time of 1900, if you look at the grand challenges of the day, they were looking for a food solution. And the reason why is in the late 1800s, every year, there were famines that were killing on the order of millions of people.
And so this is an example where scientists and engineers came together and they did, in fact, develop the solution. And that solution was from Haber. And Bosch, who took nitrogen from the air, were able to synthesize ammonia synthetically and thereby massively increase the production of fertilizer. So this was a 20, 30 year research journey across multiple countries.
Haber and Bosch in particular worked in Germany. Prior to Haber and Bosch, we mined fertilizer primarily in the form of nitrates from South America and Chile, and it was very expensive. And if we look at the cost, they would drop by $45 a ton pre Haber Bosch to less than $20 a ton post Haber Bosch.
And really what that did is that fueled a population boom. So if we look at the historical record. All right, I'm going to I'm getting the hang of it now, folks.
If we look at the historical record before Haber Bosch from 1650 to 1900, it took about 250 years for the world population to triple. But then, however Bosch comes around, we solve the problem of being able to feed humanity. And now we have a four fold growth in population in 100 years.
So I cite this example because of two things. One, it shows you that when science is well funded and we tackle the problem with all of our might, we can be successful and we can fundamentally change the way the planet looks. But also all those people that the world now holds. I was at the Davidson Academy today and I asked the students there how many people are on earth, and they immediately told me it was more than 8 billion. Now that is because of Haber, Bosch, and they're all using energy.
So all that energy that they have used now has created the current grand challenge that we're working on, which is climate change. So if there's five big picture messages that I'd like to leave you with, the first I've already mentioned scientists and engineers solve problems, but sometimes we create new ones. The good news is that history shows that science can respond in a rapid and focused way when it's resourced. And examples I'll give you will be the Manhattan Project in the 1940s, the moonshot in the 1960s and the Human Genome Initiative in the 1990s. All examples of big scientific endeavors that fundamentally changed the course of humanity. I will argue that climate change is the defining challenge of our current era, and we collectively will be judged by history based on how effectively we tackle this problem.
One of the solutions is negative emissions technologies. So here's another acronym that I'm going to throw at you net negative emissions technologies that I'm going to use throughout the talk. There's no single technology that's going to solve our problem. It's going to require innovations across the array of different spaces. One of those nets that I think is very important is direct air capture.
The reason why it's important is it's essentially infinitely scalable. So a lot of the other nets that we can use that I'll tell you about are excellent. And they're low cost, but they don't scale very well. And we've waited so long to envision CO2 as a pollutant, like we would envision other things that we would spill into the soil, water, air that we now have literally 100 plus years of CO2 in the atmosphere that we have to clean up. So we need to do two things.
And you would have heard about this in the first talk. I'm going to touch on it again briefly. The first is to reduce carbon sources.
So this includes nuclear, wind, solar, more efficient devices and point source carbon capture and sequestration. So going to large point sources like oil, coal or gas fired installations, power plants, refineries, capturing CO2 from those that produces a product that we're going to call avoided emissions. If we imagine filling a bathtub with CO2 where the bathtub is our atmosphere, this is slowing the rate at which we're putting more CO2 into the bathtub. But then in parallel with this, we need to have negative emissions. Let's first pause to think about what avoided emissions in the form of carbon capture. Looks like so we're going to do see okay to capture from a point source that's represented by the CO2 barrel in the center of the slide.
If we couple this with geologic storage shown here, we are going to have carbon capture and sequestration. The technology exists for us to do this today from point sources. We have been doing this in the oil and gas industry for decades. And if we do this on a wider scale, it's going to slow the pace of worsening climate change. But just like switching to wind and solar now is not enough.
Carbon capture from point sources is also not enough because we have to deal with those legacy emissions that Netts can in fact address. So enhancing carbon sinks is the deployment of negative emissions technologies or nets. And I was fortunate to be part of this panel in 2018 that published this consensus study report on negative emissions technologies using land based methods. And this is a fundamentally different product from avoided emissions.
Here we're talking about negative emissions technologies and one of these negative emissions technologies that's very important is direct air capture. So what are these negative emissions technologies, these things that take CO2 out of the air? Well, there are an array of different technologies, some of which you might not even think of as a technology. They're really land management approaches. So how you manage your coastal ecosystems, where carbon is stored, sometimes referred to as coastal blue carbon, or on the right hand side here, how you manage your forests and your farmlands such that you can store more carbon in soils. These are great approaches because they leverage natural processes.
They're also typically very cheap. They could be ten or 20 or $30 per ton ways to cap, to capture and store CO2. However, they don't scale very well and as I'm going to show you in the coming slides by mid-century, we need to get ten gigatons of CO2 out of the atmosphere every year going forward.
So to get a feeling for how big that is, that's roughly one quarter of our greenhouse gas emissions today, which is on the order of 40 gigatons per year. Every year for the rest of this century, starting in 2050, we've got to be taking one quarter of that amount out of the atmosphere. And that's a massive number. And these natural approaches don't scale to make that possible. But the approaches that are in the red box, including working on different ways of doing carbon mineralization with the appropriate types of rocks or taking chemical processes that take CO2 out of the air via direct air capture effectively are infinitely scalable. However, they have scientific questions that are not well, well resolved in the case of mineralization or cost questions in the case of direct air capture.
So direct air capture today costs about $500 per tonne. If you go and talk to the startup companies, the target to actually make this a viable part of our energy ecosystem is to get that down below $200 a tonne with a target of reaching $20 per tonne I'm sorry, $100 per tonne. As soon as we can. So this is probably similar to a plot you might have seen in the last lecture if you came to the last lecture. If we consider how large is the potential market for nets likely to be or equivalently how much carbon is needed to meet the Paris Agreement goals? You can see that summarized on this slide.
So this is a plot of emissions and gigatons per year is a function of time and we are basically at the apex point of this red line. And if we continue our business as usual and we make no changes to our activities, we'll hit a maximum amount of emissions sometime in mid-century and we'll certainly warm to three or four degrees C warming, which could be catastrophic for certain regions around around the world to get to something that's near two degrees C or below two degrees C, Instead, we would have to follow a line that's represented by that red line. And that red line crosses to zero emissions late in the century, but also includes bringing on a new type of technology shown in blue at the bottom. So this new technology, these negative emissions technologies start around 2030 and by mid-century rates reach about ten gigatons per year and by later in the century, reach about 20 gigatons per year.
And we can see that that's a huge, huge ask to create a new industry from nothing that is roughly 25% the size of the 2020 fossil fuel industry, which we developed over 120 years. We want to do that in 30 years. That's a huge ask, starting from a nascent state that we're in today. And so that's the challenge that we we need to undertake if we want to effectively control our climate.
We're not going to do all ten gigatonnes by direct air capture or 20 gigatons by director capture. This is going to be five or ten different technologies. But to get to a number that large, something that's scalable like director capture is going to need to be a component of it. So let's think about the act of taking CO2 out of the air. As you might imagine, this is something we already know how to do. Right.
We've had submarines for almost the entire 20th century. We've had spacecraft and space stations for the last 50 years. So we know how to take CO2 out of the air. There's technologies that exist that do that quite well. Those are pretty high cost tolerant industries. Right? The Navy's willing to spend a lot of money to keep their sailors safe.
Same with NASA and their astronauts. We're not talking about dealing with that level of cost as as as a requirement. We have to be much, much cheaper than that. So the thought to first do this as a way to combat climate change really gets credited to class Lackner, who's now at Arizona State. At the time he was at Los Alamos, he proposed large scale director capture as a climate change mitigation methodology and for about ten years, not much work was done.
There was a little bit of talk amongst academics in the literature. But by the end of the 20 aughts to 28 to 2010 was the first temperature of vacuum swing adsorption, direct air capture companies that were formed. I was collaborating with one of the first three companies that did that called Global Thermostat, and so in 2008, 2009, Global Thermostats process was married with some sorbent that we developed at Georgia Tech. About 2010, I went to DOE and ETL and advocated for funding in this space as an emerging area of carbon capture.
It wasn't really viewed to be a viable topic at that time because it was so new. Indeed, it had only existed for ten years and very few people were working on it. We wrote the first review in that area on that topic in 2011 and then further in 2011.
An American Physical Society report came out that said, Stop, stop the presses. Don't do any of this. It's going to be impossible. It's not a good idea. And I don't agree with that decision from the American Physical Society report, although you can clearly understand how they arrived at that decision. If you if you read their if you read their approach. So it took about five years for additional R&D by many groups around the world to build up a comprehensive dataset. In the letter in the literature that I think was sufficient to convince people that this in fact could be a viable technology.
And then in 2018, the negative Emissions National Academy study that I was a part of really set the tone for all of the funding that we see coming online today. And so Georgia Tech has played a really big part of this. So we got involved in the very early days. And if we look at the most recent review, which was published in December of last year, which now is up to 550 references on direct air capture, 20% of those are from GTI researchers or from Georgia Tech alumni. So we're really proud of this area of development at Georgia Tech.
So I co-wrote the direct air capture section in this report with Jen Wilcox, who's a very important person in the US Department of Energy. She's now the principal deputy assistant secretary for the also for fossil energy. And I point this out because it's really important that STEM experts go and do policy as well. And so we're all lucky that there's someone who is like us, a chemist or a chemical engineer who knows the hard science and engineering, who's now doing the necessary policy work in order to champion net funding and net deployment deployment as part of federal policy. And so it took about ten years of lobbying and convincing. But now we have multibillion dollar programs coming out of DOE.
And so for those of you who are working on new areas yourself, and if it's taking a long time for things to get traction, just keep working at it and working at it. And if it if it is in fact, an idea that merits some traction, there is hope that funding will come. So we have developed a center at Georgia Tech that we've named after the physicist Paul Dirac.
Probably most scientists know this name. Dirac was actually one of the first physicists to think about the thermodynamics of dilute mixture separations. And so he's actually a suitable person to name the center after as well as we can make a nice acronym based on his name. And every academic knows that the most successful centers are those that have the best names and acronyms.
Of course, that's suggest. So there's a number of folks who I've had the opportunity to work with who are now leading in this space. Many of them are based out here on the West Coast, Global thermostats R&D labs are in Brighton, Colorado. Miles, Stef and Eric are all working at Global Thermostat. Simon Pang is a leading CCS researcher at Lawrence Livermore National Laboratory, an alum of the University of Colorado who did a postdoc with me.
And then Göteborg is former ExxonMobil person who ran their direct air capture program as part of the team of people that excellent, who did that, who's now working for Repsol in Spain. And so very happy to have those folks out there working on these materials. So if you've never seen a gas separation process before, I have a simple cartoon that I want you to look at to understand what exactly we're doing when we capture CO2 from the air.
In this cartoon, we've got exhaust from combustion or from from the air that we're going to go ahead and pass through a contactor. And that contactor is going to contain either a liquid or a solid material that's been engineered to selectively bind CO2. Remember, CO2 in air is .04 percent of the air. So you really need to go in and pick out those individual molecules. So red is representing CO2. All of the non CO2 in air or flue gas is represented by the green.
And so we'll go ahead and pass that through to suitable contactor. It'll occur at low temperature. That contactor will then capture the CO2 on the sorbent material and we'll admit from flue gas, maybe exhaust with 90% of the CO2 removed. And so this is the same for flue gas capture at a point source and for direct air capture. CO2 adsorption is spontaneous and exothermic, so we don't have to do anything special to make this happen. We just have to contact the air with the sorbent.
The expensive step in this carbon capture process. It's the regeneration step. The regeneration step is endothermic.
And that's where we have to put energy and money into the system. So both the AC and post combustion capture are essentially the same from the adsorption site. Okay. And then so then we DS or by bringing heat into the system or vacuum or some acids are not very enthalpy fully favored. So you don't get a very large surface coverage of these carbon thick acids.
But if you keep bringing more and more amines and higher and higher density, eventually you get carbonic acids that are close enough to a second amine as shown on the bottom. And when you get that second amine in close proximity, you can deprotonate that carbon mek acid and then you form an alcohol ammonium carbonate where you have a carbonate attached to one amine. And the second amine is a base that accepts a proton and this is very heavily and thickly favored. And so the high uptake capacities that we get, we can see from this non-linear factor with the coverage of of amine sites as well as from in-situ air spectra. Copy is associated with forming these alkalinity alkyl ammonium carbonate. We can now turn our attention then to the heat absorption associated with each of these and we can see that at low amine coverage we're essentially only doing phase resorption at intermediate amine coverages.
We're mostly capturing in the form of carbon thick acids and then we can get relatively high heat absorption on the order of -95 kilojoules per mole. If we get a high enough, a density of a means to give us alkyl ammonium carbonates and then we get a very high uptake and a steep absorption curve, which is what we need for practical application. So now we have additional design elements, right? So we know we want primary means, we want to have a high density of primary amines. We can then go ahead and test the hypothesis I showed you earlier, and that hypothesis I showed you earlier was that primary means are more effective than secondary means in this case, the methyl amino proposal. And we're going to add in one more secondary mean the end butyl amino proposal.
And the hypothesis was that the bread was more effective than the blue because it had a stronger driving force for absorption in the form of a more negative enthalpy of adsorption. Well, if we look at the heat of absorption for all three of these materials, even though they have different shaped isothermal, the kind of absorptions within error are the same. So what we calculated with what we were taught in our undergraduate thermodynamics class turned out to be wrong. In this case, we can't ascribe the improved performance of the primary a means to in thought big reasons. And if it's not enthalpy, then of course, what's it got to be? It's entropy.
And the way we rationalize this is most absorbent materials are rigid fixed surfaces. A carbon silica in alumina. And so the entropy of the sorbent really doesn't change at all during the adsorption process.
But our materials have dynamic, binding sites. We have a means tethered to the surface that are moving all over the place. And in order to form an alcohol in carbon, you've got to have one amine that grabs the CO2 and the other amine that's nearby to grab a proton.
So this is a cooperative interaction. It's a two amines capturing one CO2. And in order to do that and entropic constraints can be a real problem. So when this is a primary amine, getting this nature out of the way so you can form this alkali ammonium carbonate is relatively easy. But if this now becomes a second amine chain, a methyl group or a butyl group, there's now an in tropic barrier to getting that out of the way such that you can find the the second amine to form the alkyl ammonium carbonate.
So there's this entropic barrier that a secondary amine has to get around in order to form the alcohol ammonium carbonate. And that's why we think primary emissions are more effective in secondary means. So now we have a bunch of design elements that we can go to use to design what we think might be more effective amine molecules. I've had a variety of different folks in my group working on this over the years, so we know we want primary means, we know we want them in high density and we also want them to be very stable. How many people are chemists or chemical engineers who've worked with amines in their labs before? Can you raise your hand? And do they last forever in your in your chemical safety cabinet? Do they stink sometimes? Do they degrade by oxidation? So one of the other problems we have is we have to make sure we design amines that are as stable to oxidation as possible because we're going to be using this out in the air, right.
Exposing it to ambient conditions. And so PDI is the benchmark that everybody uses because it's commercially available and it works well, but it oxidizes slowly. Pi is more oxidative, robust and we've made it in both linear and branched forms and we've made PI and PDI versions where we have aromatic groups in order to space out the amines. We've put amines on the backbone of linear polymers like polygon settle to mean as well as simple radical polymers based on poly, all the mean, all of which work reasonably well compared to the parent materials. Okay, so I did my deep dive on number two, which is designing the binding site.
I now want to talk about three, four and five as we work our way towards the end of the presentation with essentially only one slide I'm on on most of these different topics. So we've got an absorbent that can effectively grab CO2 effectively. Now we need to cycle it on and off as quickly as possible. And if we we can do that in part by having this high, high flow, low pressure drop contactor, because as I told you before, one tonne of CO2 removal at 70% capture means we have to process 3500 tons of air. So in a lot of our lab scale testing, we'll use a thermal gravimetric analyzer.
This is essentially a balance that's in a furnace and we can measure how long does it take to absorb a certain amount of CO2. This is a normalized capacity where one is pseudo equilibrium. So you can see that for air, which is 400 parts per million, we would stop after we exit the quasi linear regime of the uptake, which in the laboratory occurs after about 100 minutes. So 100 minutes is too long for a practical adsorption cycle in industry. But that's what we get in the TGA with the non optimal flow patterns.
We would not take this out to pseudo equilibrium because all this time wasted getting only a small amount of uptake would not be wise. Instead, we stop in this regime here where we get to say 80% of the equilibrium uptake after 100 minutes. Well, that same 80%, if you put it in a good contactor, that's not a TGA. Instead of taking 100 minutes, takes on the order of 15 minutes. So if you put it in a monolith, contactor like shown here on the bottom right, you can get absorption in a 15 minute cycle and then if you have a rapid way to heat it, you can get desorption even faster. And so the startup companies are talking targeting roughly a 20 or 30 minute cycle whereby you would do absorption for 15 minutes and desorption for about 5 minutes.
So how can you get that rapid heat transfer necessary in order to get the CO2 off as quickly as possible? So I mean, absorption is going to be going to occur at ambient temperatures, typically -20 to 40 degrees C depending on where you are in the globe. And then you want to have a small delta T for regeneration on the order of 40 to 80 degrees C And there's two preferred modes that you can use to deploy that rapid heating. One way that we prefer at Georgia Tech is direct stream steam stripping and the second way is via a dual heating. There's obviously a lot of other ways you can do it as well, but those are the two we prefer at Georgia Tech. So steam stripping with saturated steam is one of the fastest ways you could do heat transfer. Heat of condensation of steam onto a sorbent material can give you very, very rapid heat transfer.
So we'll take our sorbent, which if you remember earlier, was yellow with trapped CO2 in the form of red. We're going to go ahead and pass steam over. That steam will condense on the surface, and it'll provide two driving forces for desorption. One is the heat, the rapid heat conduction into the sorbent based on the steam condensation. And the second is the change in partial pressure, right? So now we're diluting the the concentration of CO2 over the material. Once we have a steam CO2 mixture, that's a relatively easy mixture to separate by condensation.
You can condense out the the steam, recycle it, and then you have essentially a pure CO2 stream. This requires a very robust sorbent. So for those of you who remember what I talked about earlier, for my sorbent, I in the lab, I use a lot of silica and a means a silica and an amine and steam makes mush.
So that's not what you would do at scale. You got to use a more robust metal oxide. And so we typically would use an aluminum silicate or a alumina that can withstand that sort of steaming.
The second approach, what we've developed more recently, and this is really due to the expertise of my colleague Ryan Lively, is to do dual heating. So we all hear about how we're going to have all this free electricity at certain times of the of the year when renewable energy is so efficient. I don't really believe that, by the way. But it's nice that we have low cost electricity. And if we do and if we want to use electricity for desorption, we can do that by joule heating. So rather than making a polymeric fiber with silica particles in it, we can take a carbon fiber coated with our sorbent layer.
In this case cellulose acetate with silica particles put amines in there and then we can rapidly heat from 20 degrees C to 120 degrees C in a matter of a minute or less by joule heating by putting a voltage across that carbon fiber. And compared to a typical temperature swing adsorption, which gives you a broad curve of CO2 that's released, you can probably barely even see the blue curve in the back. The red curve with electric swing adsorption is a very sharp pulse so we can get a very high concentration of CO2 off very quickly with joule heating. If we have a sorbent that's robust enough to heating up to say 90 degrees C and and then cooled back down very, very rapidly. So you've got to have a way to rapidly heat and cool in order to cycle this material quickly.
So the last element number five on the list is acceptable capital cost and ultra long process material lifetime. And here sorbent degradation in lifetime is a critical element. So amines are not going to last forever in an exposed to atmosphere, certainly not with temperature swings involved. So I've worked with a start up company that I told you about earlier Global Thermostat. They originally had their R&D labs at Georgia Tech and then moved them in 2020 during the pandemic to Brighton, Colorado. The Georgia Tech lawyers require me to give this conflict of interest statement that says that I receive research funding from them.
They've licensed IP for me. I have a financial interest in the company so that if ever they ever make $1,000,000,000, I'll make $0.50. And this is a first generation unit. They built in Menlo Park, California in 2011 at Swri. This is the third generation unit that was built in 2018 in Huntsville, Alabama, and the fourth generation was is being unveiled in March in Brighton, Colorado. So what is the process look like for one of these direct air capture approaches? We've got five steps in a cycle and we do adsorption in the first step.
We would then close off the steps the system to airflow and evacuate it to remove interstitial air around the absorbent materials. We can then inject steam into the system, heating up the system and dissolving CO2, collecting the CO2, closing it off again and cooling it back down to a suitably low temperature, at which point we can open it up to airflow again and continue to absorb CO2. So we've done a techno economic analysis and published it in the bottom paper here with my colleague Matthew Ralph, who is a systems engineer.
We used a MOF containing sorbent mill one on one chromium containing PTI on the monolith contactor. We did that because we had to use something different from what the startup company does, and we can calculate the cycle time, the energy used, and the energy is about 0.15 mega mega joules per mole CO2, which is above the thermodynamic minimum. It's always good to check to go ahead and check that we intend to try to scale out this technology by mass manufacture.
So one way that companies are envisioning deploying this is with essentially shipping container sized units that would be paralyzed and deployed across across the country. And the biggest unknown is the lifetime of the amine sorbent. And we know that amines are not stable indefinitely in air. So we've also spent a lot of time studying the oxidation of amines and understanding the mechanism of oxidation and how we can inhibit that. We can go back into the old literature and see that it's all of our data are consistent with with with what's referred to as the basic auto oxidation scheme, which is a radical abstraction of C-H bonds of a polymer. And we've recently published a paper on the chemical kinetics of the auto oxidation API, along with the start up company calculating a reaction order and O2 of about 0.52.7,
and an apparent activation energy of 105 kilojoules per mole for the oxidation. The degradation of these amines. And so using these kinetic parameters, we can now extrapolate how long amine formulation will last in the field based on how it behaves in the laboratory. And the way it degrades is we're seeing an increase in the carbon nitrogen ratio under all conditions.
That means we're breaking carbon nitrogen bonds when it degrades and we're seeing a reduction of the H over C plus N elemental ratio, which means we're also breaking C h bonds. So these radical reactions are attacking C-H bonds and C bonds. And so this alpha carbon next to the the nitrogen group is a is a relatively acidic C-H bond. And that bond can be abstracted.
We can react with oxygen to create OXO or hydro peroxide type intermediates, and we can draw different pathways that produce amine groups that produce carbonyl groups and then ultimately that give off ammonia. And CO2 is the final products for degradation, all of which we have detected in the laboratory. And so we're getting down now to the level where we're beginning to understand the elementary steps of these degradation reactions, but then which then should allow us to design a more robust sorbent since something as simple as putting one more methylene between the two nitrogen goes a long way to making these materials more oxidative stable.
Okay, so all five of these elements we've demonstrated on the in the lab, there's a couple that have the most room for improvement. I think those are four and five, minimizing the energy of desorption and increasing the lifetime of the sorbent materials. But I'm going to finish up with what I think is the current cool topic that we're working on in my group, the six critical element that really not enough people are talking about, and this is the technological flexibility. So this is a technology that we're going to optimize differently from almost all chemical technologies that we might have dreamt of. So if you imagine a chemical technology whereby you're going to create convert crude oil into naphtha and intermediates for chemical synthesis, polymer synthesis, we can optimize that in our laboratories wherever we're working, and then we're going to deploy it under the same conditions in in France, in Brazil, in Saudi Arabia and in Texas. Right. We'll optimize it for that one set of conditions.
And within a little bit of wiggle room, we're going to operate it under those conditions. Direct air capture is definitely not that you're going to have a changing feed in real time. It could be raining in the morning, hot and sunny in the afternoon.
It can be winter, it can be summer, it can be think about what January in Reno is compared to what July in Reno is very, very different. And then even in a single day, you could have five degrees C or and then 25 degrees C later in the day. And your process has to work under all of those conditions. And so we are probably going to imagine a process with real time sensing of temperature, relative humidity, and you might be have a process where you're changing the cycles in real time, depending on the weather.
And what this means in my view is that you're not ever going to have a single DC solution that dominates everything because I think there's going to be different solutions that operate in different climates. So a DC solution that might work in the Reno climate is not going to probably work in the Miami climate. And so there's real opportunities for many different types of innovations to be actually deployed and used if you can optimize it for a certain set of conditions. So if we look at the average temperature around the world as a function of location, we can see that there's definitely hot zones.
And if you look in the literature, all of the data on DC are in this temperature region that I would call ambient and it makes sense, right? So ambient I'm defining is starting at 20 degrees C, that's indoor room temperature. That's where our labs are. Of course, that's where we're going to be taking data. But actually, if you look throughout the year in the different regions in the US, south, southwest northwest, a large fraction of the year, the average temperature is below 20 degrees C And in this region there's almost no data in the literature about what types of materials work well and what types of materials don't work well.
And so I think there's a huge opportunity to work in this region. And in particular, I think the most promising region for DC is actually working in colder climates and for me, the optimal place to deploy would be somewhere like Calgary, Alberta, in Canada, where you have a workforce that understands chemistry and chemical engineering, you have geology that allows you to store CO2 underground and you have the driving force of cold temperatures driving adsorption. So we can think about working in cold temperatures. And in fact, it might actually allow you to use technologies that we previously thought wouldn't won't work at all. So remember,
I started the talk on soybeans telling you why Zeolites won't work. Zeolites are fizzes orbits and they have a hard time distinguishing between water and CO2. So if you have a humid day at 25 degrees C, you have almost 32,000 parts per million of water in the air. And if you have that much water and only 400 parts per million of CO2, capturing the CO2 and not the water is going to be a huge problem. But if you go to cold temperatures, say -20 degrees C, you're now down an order of magnitude in water content even at the at the dew point. So we're down to about 1240 parts per million.
So I think cold temperatures and climates offer many possible advantages, including favorable thermodynamics for absorption weaker, which enables you to use weaker CO2 binding. If you have weaker CO2 binding, it's less energy to regenerate the sorbent and most importantly, less competition from water. So we a simple study, just a thermodynamic calculation. By one of my second year engineering students, it was a good opportunity for him to do some very basic work. Let's imagine we're capturing CO2 from air at 25 degrees C using ZEOLITES and it's a humid day. The amount of energy it takes to regenerate the water off the sorbent compared to the amount of CO2 that you capture is way higher.
Maybe a factor of three or four higher just to get the water off the sorbent compared to the CO2. But if you do this at cold temperatures because there's so little water in the air stream now, you might be able to be able to use two beds. So we could use a desiccant bed to remove the water and then a second bed of zeolite to remove the CO2. And we think at cold temperatures you can use zeolites. And why is that important? Because Zeolites have an existing supply chain that you can go and buy now and deploy now.
A lot of those fancy amines that I showed you earlier that we spent years trying to make, they work, but it's probably going to be ten years before I can get a company to make them on the ton scale. Zeolites You can go buy on the ton scale tomorrow. Just bring your checkbook. So to give you an example of how the different temperatures and conditions matter, I'm going to give you this last little element and this is where we used a mil one or one mof with amines in it. And previously we studied at 25 degrees C and showed that they captured CO2 very well.
And I just asked the simple question, would it be different if we now deploy this material at -20 instead of 25 degrees C? And so here's a plot of the capacity in moles per kilogram as a function of amine loading. And at 25 degrees C, the performance is that it gets better at higher amine loadings. Remember the nonlinear performance of amine site density with respect to CO2 uptake, the more amines you put on, the better it behaved.
That's why we have this concave shape as we go to higher amine loadings. But now if we go to -20 degrees C, we have a totally different behavior. You get an optimal performance at a lower amine loading which is below what you get at 25 degrees C But the take home message is that you would use a different sorbent in the winter from what you would use in the summer if you were to deploy this family of materials. And this is why I think climatic zone is going to be important going forward.
So I'm going to finish by answering the two questions that everybody always asks me so that therefore no one will ask me. The first one is how much land do we need to do this? So I've heard other energy technologies that are really land intensive. And so I did a back of the envelope calculation. So let's imagine that we do the 2060 negative emissions target for the whole globe. So all negative emissions that the globe needs by AC. I'll begin by saying I hope we never, ever do that because the AC is the most expensive net and if we do the whole world's de ac work with the whole words network with the AC, we're all going to be a lot poorer.
But that would be ten gigatons per year if we were to do that. Based on my connection to the start up company about the land area that's required per tonne of CO2, they estimate that total global need would be about 152,000 square kilometers of AC implementation. That is roughly the size of the state of Georgia. So I'm not arguing that that's a small amount of land, but I'm saying that if that's what we had to do somewhere on the globe in order to stabilize our climate, we could do that.
If you consider all the landmass and the wasted landmass that exists around the globe, this is not an intractable amount of land. And I'm not volunteering Georgia for this because I quite like living in Georgia. The second question I get is why not use natural systems like trees? And my first answer to that is that as a as a species, we have not demonstrated the ability to reforest only de forest.
So until there's empirical evidence that shows that we can reforest and a little bit doubtful that we can do this as a as a society, but let's say even even if we could, there's there's something to be said about the direct air capture approach. So let's take a best case scenario for not a best case scenario, a good case scenario for a tree. We'll take a tree at the equator.
We'll go into the appropriate literature and we'll calculate the flux of CO2 removal from a tree at the equator, and we'll normalize that by the ground area. And if you do that, you'll get a number that's on the order of 20 micro moles per second per meter squared of ground area. And then you can do the same calculation for a de ac process and you get a number that's 25 times higher. So from a land use perspective, these de ac plants are much more efficient than a tree at the equator. Now, I'm not arguing that they're better than a tree at the equator.
I would much rather have us do as much of this problem as we can by planting trees and doing natural things. But we can engineer systems that are more efficient per per square meter of land than than biological systems. So the technical summary then is that direct air capture is a key scalable net. And what makes it important is that it's scalable and. Watts it makes it not deployable now is it's way too expensive.
It's on the order of $500 a tonne and we need to get that down to 100 or 200 an element of this technology is a low pressure drop, contactor with high capacity sorbent with rapid kinetics and a small delta t Those are design elements of the process and you need to design the sorbent the contactor in the process together. This is not a technology that I think is going to work well by materials. People working in isolation from process, people they have to work together.
One of the big problems for the sorbent that I have worked on mostly, which are amines, is degradation by oxidation that has to be managed. But if we can go to low temperatures and use purely inorganic materials like physics orbits, those will be essentially, by comparison, infinitely have infinite lifetimes. And then they're just overall lack of data at the cold temperatures and in varying humidity where there's a lot of fundamental science that remains to be done. So these were the five points I started with. The last two are important is that nets, along with renewable energy, are going to be the answer. One net, not the only net is AC.
I want to acknowledge my senior collaborator is on the left hand side of this slide. My former students who've worked on the AC, my former post-docs who've worked on the AC, those who are bolded are people whose work I talked about today. They're working all over the country in the world now on this topic and then current picture of my group, those who funded me up for carbon capture on the right hand side, and any of you who are interested in getting, say, middle school or high school students interested in a STEM career, I partnered with the Dreyfus Foundation to do a seven minute video on DACA and how it can be a society changing, climate changing technology.
You can find the link to that, that short film on that link right there. And so with that, I'm at the point where I'm happy to take any questions. I hope people will stick around for a discussion.
It would be really nice to have opportunities for us to all interact and ask questions and I'm happy to stay as long as necessary to answer as many questions as they are. So I really appreciate everybody's attention. Sorry that I didn't know how to use a remote early on and thank you again.
2023-03-31 10:23