US-MAP Webinar — Perovskites at the Edge of Tomorrow
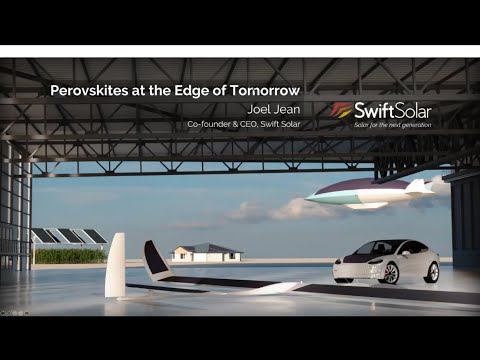
>>Joel Jean: All right. Great. So yeah. Thanks Harrison. Thanks Laura and Joe for inviting me to speak today. Again I’m one of the founders and CEO of Swift Solar which is working on bringing perovskite tandem solar cells to market. And I imagine that today we have folks from all kinds of backgrounds in the audience from students to perovskite researchers to investors. So please bear with me if I’m telling you things that you already know. I’m sure I will. So anyway, our mission at Swift is to build a world where all energy is clean energy. And this has been true since the very first meeting between the
cofounders. We talked a lot about hopes and dreams. And it was pretty clear that what we all wanted was first to help fight climate change and second to build clean energy projects that serve the people who need energy most. So that’s why we started Swift. That’s how we built the company and the team and that’s where I want to start today. As a society we need to deploy lots of solar PV because we need to do something about climate change. And we also think of climate change as some far off problem for some island nation sinking under the sea or for our grandchildren to deal with. But it’s an existential risk
today So almost every major problem we face in the world today is either caused by or amplified by climate change. Everything from heat waves to power outages to pandemics and refugee crises. They’re all linked to climate in some way. So climate change got real personal for a lot of us in 2020. This is a photo I took last September from my front door. And the orange sky, the raining ask are all caused by these massive wildfires that we saw on the entire west coast and even in Colorado. And these wildfires are very closely linked to about 150 million trees that have died in California since 2013. That’s probably because of drought which weakens the trees and
makes them more vulnerable to things like bark beetles which tend to hatch earlier and reproduce more frequently in these warmer winters that are caused by climate change. We also see an increase in lightning strikes which are a major cause of dangerous wildfires. And we expect that with the higher temperatures we’ll get even more thunderstorms and lightning. Something like 12 percent per degree Celsius of warming in
the US. And just overall we’ve seen that the frequency of days in California, fall days in California with extreme fire weather has actually increased by 2X since the 1980s and it’s only going to keep getting worse. This is also true for COVID actually. So infectious diseases by definition involve interactions between species, right, a host and a pathogen, sometimes risk factors in between. So infectious diseases rise when humans come into closer contact with animals
which happens when habitats are destroyed by droughts or wildfires or flooding or anything else from climate change. We also see diseases rise when vectors like mosquitos and ticks spread. And that includes when high temperatures increase their metabolism or when increased flooding gives them more breeding sites. And diseases also rise directly people are more likely to get sick which is what we see with more extreme temperature swings and warmer winters which actually reduces herd immunity in subsequent years. And the third thing is that these impacts aren’t
actually isolated. So extreme weather can also increase infection rates and so on. So with all that said, what are we going to actually do about climate? And the easy answer is to stop digging, right? Stop drilling, stop fracking, stock burning fossil fuels and start using other energy sources that don’t emit carbon. You all know this already. And it turns out that solar PV is a pretty good way to get zero carbon electricity. So here we’re showing the life cycle carbon intensity in terms of grams of CO2 equivalence per kilowatt hour for all the different electricity generation technologies we have. And
obviously coal and gas are not so good. Gas is generally better. But if you account for methane leakage it’s probably not that much better. And certainly with a whole array of possible low carbon technologies including PV which does pretty well. It’s about life cycle right now about 50 grams of CO2 per kilowatt hour. Thin film is a bit lower so
that’s just silicon thin films are maybe more like 20 to 30. These will only go lower as we decarbonize the grid. Sunlight isn’t very energy dense so you need lots of land. But it’s actually less than most people think. So a lot of times when we think of covering whole states with solar to power the whole US but it is in fact – this nice figure from my friend Patrick shows that we only need this little square. It’s pretty small,
right? So this little square to satisfy all of the US electricity demand with PV. And that’s with average efficiency. So if you actually go ahead and look in Arizona you need less. So this is a good amount of land but it’s not that much especially if you’re looking at comparing this to the amount of air we use for coal mining which is right about the same or even combining missile testing ranges and golf courses you kind of get the same amount of area as well. You only need about 50 percent of the area we use for corn ethanol. So all these suggest that there are a lot of uses of land that we could easily replace with solar. And that’s doing pretty well on the
climate side. Obviously we’re not looking to go 100 percent solar. That’s not optimal choice for the grid and the land isn’t going to be limiting. And on top of that everyone has access to sunlight globally. So obviously, right? So here we’re showing the solar density instances, megawatts per square kilowatt. This is just a measure of density on the same scale as on the x axis for uranium and oil. And these are at large scales but on the same scale. So you can see that solar varies by about a factor of
four from the sunniest country which is _____ and the cloudiest which is Norway. Right? So if you look at other energy resources like Uranium for example you have a thousand x variation between countries or oil where you have a million x. Those are way less equitably distributed. And probably most importantly solar PV has already been used at large scale. It's affordable compared to fossil fuels. We've seen that PV is actually on track to surpass
wind in the global installed capacity in 2021 with over 600 gigawatts already installed. And about ten years ago I mean terawatt scale solar sounded super far away. But now we're almost there. So PV has gone from super expensive to super cheap in the last 40 years dropping from about $8.00 a kilowatt hour in 1975 equivalent which is something like a utility bill of $7,00.00 a month for a typical household in the US to less than 10 cents a kilowatt hour in 2020. Where it's now the most affordable source of electricity for
a lot of the world. So LCOE obviously isn’t the whole story because sunlight is variable and intermittent. But the cost of storing that energy, that excess energy from when the sun is out with lithium ion batteries is also coming down at an insane rate. So because of this huge progress in solar PV in the last ten years that a lot of people will now say solar is solved. Right? Solar has won. We should inspect our work on agriculture or cement or steel or electric aviation or fusion or something else. And obviously everyone wants to work on and invest in the more unsolved problems. So that's the dominant narrative. Solar is solved. And honestly I think it's true for the
most part at least on the surface. This wasn't obviously true ten years ago or even five years ago. But now there is good evidence that we can likely eliminate about 90 percent or more of power sector emissions without a whole lot of change in the average cost of electricity or using just existing technologies, things like PV, wind, hydro, lithium ion and new transmission. And there's some nice work by _____ and others that shows that
you can scale up renewables and electrify everything using EVs and heat pumps and batteries. And that alone can actually eliminate something like 70 to 80 percent of US emissions by 2035. So that's with no new technologies. So today I actually believe that silicon is probably good enough. We don't need to wait for perovskite or other new technologies, PV technologies to solve most of that climate problem and that's actually really good for the world. But personally I also think it would be a huge mistake to stop innovating in PV technology. Just about every single low
carbon pathway, every integrated assessment model, every power system model that I've seen relies heavily on PV to lead the way in decarbonizing the power sector. So that means that even a small change in LCOE can make a huge difference. Right? The 10 to 30 percent savings you might get from making PV more efficient and easier to install could translate to on the order of $100 billion of value per year in the US alone. But
today we're systematically under investing in new solar technology. So here's some data on most of the high profile US solar investments over the last 20 years or so. And a bunch of companies raised hundreds of millions of dollars from 2006 to 2012 to build the next big thing. Right? And if you haven't seen a bubble freeze this is what it looks like. After Solyndra blew up in 2011 after over a billion dollars raised US investments in solar PV basically froze for the rest of the decade. So I've seen this first
hand with doing fundraising where I have had VCs say that their LPs won't let them invest in solar after Solyndra. So this is clearly a barrier. This kind of rise and fall has been a barrier to continuing to fund new solar technologies. So in the last decade solar startups in the US weren't really getting funded. But in the background obviously technology was still improving steadily. So perovskites this new
technology has now surpassed every other thin film in small area cell efficiency. But a lot of - there's been very little private funding that's gone to the US PV companies during the same period. So over the last three years it's only been something like $20 million in total that's gone into US startups building new and emerging PV technologies. And just for comparison in this same time period we've seen over $400 million invested in nuclear fusion. The same amount invested in waste disposal startups. So anyway my goal here is not to - obviously not to downplay the importance of zero carbon baseload power or sustainable waste treatment. But if we are going
to rely on solar to solve 20 to 50 percent of the climate problem and if we're spending trillions of dollars deploying solar worldwide in the next 30 years and indefinitely after that it seems worth it to keep making it better. So silicon is the only PV technology we ever have it's not the end of the world for climate. But we are missing out on a huge opportunity to build a cleaner and more equitable world. So as we look forward to a potential future for perovskite I think it's useful to look at why previous solar startups struggled and what we can learn from them. I think there are a few different factors worth considering. And some of these include just bad timing, to enter
the market when China was scaling up silicon PV massively. The choice to compete with silicon head on on price which forced them to scale the factories too early. So you spend hundreds of millions of dollars on factories that are running not completely proven processes which ultimately led to running out of money before finding product market fit and ultimately selling the company to a questions acquirers. And a lot of these things are difficult to control. Right? A lot of them were issues of circumstance or macro-economic
realities. But anyway, it's not a pretty picture. And we have to look at these failure learning opportunities and try to chart a different path. So to kind of walk through these, some of the learnings you might get. I mean fundamentally the world in 2020 is different from the world of 2010 for a lot of reasons.
We importantly now have some real public awareness and momentum on climate change. We know where China stands. Governments do seem to be more aware of the strategic importance of energy and domestic manufacturing. And with things like stacks
and other capital sources for better or for worse we're starting to see new pathways to both capital and assets for clean tech which was nonexistent in the first clean tech wave. We also know not to underestimate China in silicon where the targets for cost floors and efficiency ceilings are always moving. We need to outperform rather than undercut silicon and also find customers who will pay for good products and not just cheap products. And of course another option is to couple perovskites with silicon in a tandem cell. Another important learning is to develop technology and manufacturing processes and find that product market fit before you go and build and scale up your factories. And
maybe the ultimate learning, a very important learning at least is that product market fit and unit economics matter. So new PV technologies need to have products that have good unit economics. They need to have a financing plan that matches their market strategy which could include mission aligned private investors and government support. And I think it's really important to recognize the value of government support here which really should be sustained even in the face of high profile failures. One of the shining
stars of clean tech 1.0 was Tesla which some people may know funded its first factory with DOE vehicle manufacturing loans and actually have been supported heavily by EV subsidies and emissions credits. So a lot of possible learnings we can take from the last generation. So to kind of look forward I just want to start to introduce some of the new technologies including perovskites and lay out the landscape a bit. So there's many ways you can try to classify and organize the PV technologies. One way is to use material complexity as sort of a guiding metric. And this is very hand wavy but you can kind of look at a spectrum from
things like _____ silicon which are wafer based, have relatively simple materials, single atoms in a structure in a basis. But then you have very complex processing, very high temperatures, requires crystallization, doping, things like that. On the other side of the spectrum you have things like quantum dots and organic molecules which are very complex if you look at the building blocks of these materials. But they are processed in very simple ways at low temperatures. And these materials on the right side, these emerging materials including perovskites tend to be more abundant.
They tend to be more tolerant to impure precursors and disorder. So you can actually manufacture them more cheaply in many cases. And those lower process temperatures, lower manufacturing temperatures are a big advantage. So perovskites and other emerging technologies can be manufactured at below
200 Celsius, 200 degrees Celsius compared to 500 plus for silicon and commercial thin films like CdTe and CIGS. And these lower temperatures reduce your energy use. They make it easier to design equipment. For example compared to CIGS and CdTe and they don't melt a cheap and flexible plastic substrates which is helpful. So in that direction if you're looking to make a very high powered weight or very lightweight solar panel you really need to go to a very tin substrates. And
these are important for mobile or portable solar applications. And this is showing the specific power in watts per gram against the thickness of different substrates. So these are on a _____ scale. So obviously these efficiencies are a bit dated but changes don't really matter to _____ scale. In any case at the thick substrates
you obviously have the substrate is dominating the weight of the full module or the full cell. And in contrast as you go to the left to a regime where you have very thin substrates you get to a point in the limit where active layers dominate the weight. And in reality you're going to somewhere in between these. But it's only when you get to this left side where the active layers start to dominate that having these lightweight thin flexible active layers really matters. So perovskites start to shine when you go to thinner substrates alongside other emerging PV technologies.
So at MIT we did a silly thing and we put a solar cell on a soap bubble. This is obviously pretty useless. But we did this to show sort of what you could do in the limit of having a very thin substrate, very lightweight solar cell. And we were able to achieve over 6,000
watts per kilogram. So that's like a whole rooftop installation in a kilogram. Right? So quite a lot of power in a small lightweight package. This is actually an organic solar cell, not a perovskite. One more thing is that because these emerging technologies use abundant materials that are produced in high volumes they can often reach terawatt scale deployment without requiring a lot of growth in production of the raw element. So you compare silicon which needs about six years of silicon production to satisfy all world electricity demand if you were to use 100 percent silicon PV. telirium you need something like 1400 years. And
this has been kind of a dead horse that's been beaten a lot for CdTe. And I do want to emphasize that you don't need to satisfy all electricity demand to be a useful technology so don't want to downplay this. But certainly when you look at the emerging thin films on the right side you need something like less than three years for all of these technologies to generate all the materials, all the raw materials you need to produce terawatts of electricity, something like 25 terawatts with all this single technology. So that's actually really important from a macro perspective. It lets you - if you can avoid having to
actually increase the production volume of these materials. Then you're not limited by mining. You're not limited by biproduction dynamics of how these raw limits are produced. And all of those things make you a less risky technology in the end when you're looking at large scale. So the point of all of this is that emerging solar technologies do have some fundamental advantages compared to silicon in terms of weight, flexibility, potentially efficiency, abundance, cost, manufacturing. A lot of potential advantages. And anyway we're all here because we want to see these advantages realized in the market and perovskite is the most promising. So let's look at a few ways that perovskites might actually be useful in real
applications. So natural use case for perovskite in orbit in space where the SpaceX and OneWeb and others are looking to deploy thousands of satellites to provide internet connectivity around the world. So this could be tens of megawatts each year of solar and market size of hundreds of millions for the solar alone. And perovskites here have a
natural advantage because they can be lighter weight, more efficient and radiation hard it turns out than silicon cells. Another application is powering unmanned aerial vehicles, UAVs and air ships for months at a time using only sunlight. So you can do a lot of things with UAVs but one exciting application to me is you can bring internet and communication services also to remote areas or improve them. So if you have a group of people spread out in a rural area without access to a cell tower you can actually introduce the UAV or network of UAV flying ahead in the stratosphere and that can act like a flying cell tower and create a network that acts as a go between to link people to base stations. So you can think of these UAVs as a complimentary form of satellite. Easier to launch
and actually recoverable. And they're fully dependent on efficient lightweight solar. So leading aerospace companies, a lot of them are actually working at building these platforms. So it's still early days for the applications that I just describes but there's actually a lot of weight behind them. And we can help unlock this market by making lightweight and efficient solar cells in a more affordable way than conventional space cells.
One application that's actually really interesting to me is looking at solar water pumping and other productive loads in India. This is obviously coming from, coming out of the MIT Gridex Solar Program which was focused on these kinds of applications. But if you look at India's electricity about 20 percent of it is used for agriculture today. And a lot of that is for irrigation. And irrigation is super important. But a lot of the small farmers actually don't have consistent access to grid power. So solar water pumps have become an interesting way to bridge that gap.
So the Indian government has actually been able to deploy something like 1.75 solar pumps in the next few years which is almost a gigawatt market and potentially higher. And the importance here is that if you want to be able to share panels a lot of the farmers actually share the economics and avoid theft, you need portable lightweight probably rollable panels that can be moved around and set up by a single person.
And there's a lot of companies working on these kind of solutions already in India. There are many of them based on crystalline silicon today. Claro Energy, Atom, for example. These are photos taken by our Grid Edge teammates who basically saw these use cases.
And you can see that they're on wheels which is really interesting. They're often put on carts and weight naturally is a challenge here. These are human powered solar, mobile solar. So you can actually look at powering all kinds of other productive loads as well, right? Things like sugar cane or fruit juicing machines for street vendors. On the right we have electric rickshaws for short distance transport, wheat threshing, weaving, all kinds of agricultural productive loads as well. And I think learning from ______ over at MIT in particular and the Good Edge team in general I think the e-rickshaw market on the right is actually really interesting. It's a very
large market and these things are often used as a last mile connection for a few tens of kilometers per day. and you can actually retrofit them to do a lot of things. And they have a hard time getting access to power since they're often just standing in the road during the day and they're off grid. So lightweight solar can make a lot of sense there too. But going back to the US or globally we can also help open up a new class of self- charging EVs, solar powered EVs, cars and trucks and buses that charge themselves with sunlight. So a lot of people think that sunlight is not energy dense enough to power vehicles. But if you actually go through the calculations you find that if you have solar that's efficient enough you can actually drive something like 5,000 miles a year on sunlight or almost half your annual miles. So with a relatively small upfront investment, right, a couple thousand dollars, maybe a thousand dollars you can actually get a lot of convenience of plugging in your car a lot less often, the piece of mind of having that extra source of power. Even cost in CO2 savings for not
charging with grid power so I think that's pretty cool. If only because perovskite technology is flexible we can start to make solar integrated roofing products that roofers can install at the same way and same time that they install your new roof which could actually drive down the cost of residential solar dramatically largely by reducing soft costs and help make it more affordable for almost every household. So obviously this has been tried before a few times with CIGS products and silicon products. But to my knowledge it's never been done with tech that actually can be more efficient and more affordable than silicon. So perovskite has really opened up I think a new opportunity in this range, in this domain.
And especially for this market, sorry, for this audience I think it's important to recognize that these are all viable markets for perovskites made in the USA. Right? So these, the economics work out such that you can manufacture in the USA and serve all these different markets. There's a lot of strategic importance for the country I think in being able to open up these applications and deploy perovskites. Anyway, I know some of you wanted to hear, you probably showed up here to hear what we're up to at Swift. so let me quickly introduce the team and the company. So these are
the founders of Swift. We have six cofounders coming from five different countries. And we've worked together on this technology for a lot of the past decade. So several of us have been, had a lot of intertwining paths. Thomas and I were actually classmates at Stanford during undergrad and through PhDs and post docs at MIT, Oxford and Stanford. And ultimately we came together at NREL in 2018 to launch Swift. And I'll just say that my cofounders are all world leaders in the field of perovskites.
They're pioneers of perovskite tandems. And on the team we have experts across many aspects of solar technology and techno economics. And we've been lucky to add team members and advisors who have really helped push the industry forward over the past 20 years or so. So we started with 6 people. We have 18 including a couple interns and part
time folks. One thing I like to point out is that we have people from ten different countries out of 18 people which maybe just goes to show how international the community of perovskite and solar research is and hopefully how globally we can deploy solar as well. So quick rundown of the numbers. We've raised about $16 million in equity financing.
Only about $1.4 million from traditional VCs for a lot of the reasons that I named earlier. We again have 18 team members and 9 PhDs for better or for worse, a lot of PhDs sitting around. And we have four federal grants. And we've been able to use our funding to actually build out a facility with 10,000 square feet in Can Carlos, California. It’s about half lab and half office. And it's completely tailored for perovskite elements. So the design of our lab pulls from our team's experience at a lot of the leading labs, NREL, Stanford, Oxford and elsewhere. And we have equipment for making cells and many
modules for testing cell performance and testing product reliability. And the technology that we're focusing on that we believe is the future for perovskites is all perovskite tandems. So again the core advantages of perovskites are that they can be formed from these blended and low cost precursors at low temperatures but they still have this very, very high quality. right? To create high quality semiconductors at these
low temperatures which means you can make a very efficient solar cell with a thin film. And a particularly interesting property is that you can actually tune the colors by tweaking them through a composition. So we stack two perovskites up on top of each other. So one absorbs the UV in more blue light, transmits the red and the infrared which gets absorbed in the second cell. And because you're optimizing each cell for a narrower part oft he spectrum you can make a tandem that's much more efficient at converting sunlight into electricity than a typical cell.
So obviously the leading technology today is silicon. It's about 95 percent of the market. It's been in the lab since the 1950s and it's creeped up in efficiency, right? Still going up at the cell level up to a theoretical limit of about 29.5 percent. In perovskite single junctions we know came around around 2010. And our team members have been at the forefront of this really helping advance the technology to over 25 percent today. But
these single junction cells are always going to hit a ceiling around 30 percent as well. So what we find exciting about tandems but all perovskite and perovskite silicon tandems is that they've improved just as quickly and they can break through that ceiling. So they can ultimately reach efficiencies as high as around 32 or so percent in the near term and well over 40 percent eventually in theory. So this is the exact same strategy that's been used successfully with other semiconductors, specifically with three to five materials like _____. And they've reached efficiencies as high as 39 percent with fixed junctions but they also cost like $10,000.00 per square meter so 10x more than silicon. And what the perovskites offer is the same kind of performance, maybe almost as good of performance at a cost that's actually lower than silicon and that's a game changer.
So if you want to go ahead and make an all perovskite tandem you have to think about the entire stack and the entire module structure. And how do you design this? Right? So there's a lot of pieces. On the low gap cell you need to make a low gap perovskite which usually introduced tin to the material which tends to produce stability challenges. So you need to be able to make a stable and efficient low gap perovskite and you also need to make a stable and efficient wide gap perovskite which introduces bromine which can cause phase segregation and other stability challenges as well. You need a rear electrode that actually physically blocks ions from reaching the metal contact or simply just doesn't react with ions in the perovskite and other layers. And these problems usually show up
over time over the longer times. So we need to be aware of these. Maybe the most important layer in a tandem is the recombination layer which goes between the lower light gap perovskites. You have to electrically connect the sub cells with this layer by efficiently recombining holes from one cell and electrons from the other. We've had a couple of design considerations. You do need to make sure that this recombination layer isn't too conductive laterally so you can avoid coupling the shunts. You also need to block ion diffusion between the sub cells and also damage to the bottom cell during processing of the top cell. So there's a lot of competing requirements,
optimization variables here for the recombination layer and that's why we spent a lot of time working on making this a very high performing recombination layer. And finally the optics are really important here. So if you change the short circuit current a small amount in a tandem it actually has a much bigger impact for tandems than for single junctions. So we need to optimize the layer thicknesses in optics to reduce optical losses and match the sub cell currents. That's obviously true for two terminal primarily for the current matching at least. So obviously this whole stack needs to be made using
low cost materials and manufacture that at high throughput. So here's an example of a real all perovskite tandem stack worked on by _____ at NREL and two of my cofounders Charles and Thomas and many of other folks, many of our collaborators at NREL. This was made into a very high efficiency flexible cell. And really what these tandem perovskite tandems open up is the possibility of making really nice looking solar products that are as lightweight and flexible as wrapping paper with better performance than state of the art. So it's this combination again of this high
efficiency, of the lightweight, flexibility and affordability that really opens up the new opportunities for solar that didn't really exist before. So we've been heading in that direction, designing very lightweight PV products based on these perovskites. These pictures are for single junctions but certainly you could do the same thing for tandems. And a key piece of this is making a lightweight package. So you
need to again because of the substrate and the packaging dominates the weight, you need to have very lightweight packages with good barrier properties to make these very high less over kilogram products. You can see they're obviously very flexible as well. So that's great. If we want to put perovskites a bit more into context we can compare them with other PV technologies. So starting with silicon, right, efficient, very long lived, very low cost but not lightweight at all. CdTe is actually very similar.
CIGS is efficient, could be lightweight, long lived but generally has had trouble getting to very low costs. Organic solar cells efficiency is a downfall there but they can be very lightweight and have other properties like being transparent or colorful and stability or long lifetimes are still under development. III-V as I mentioned very efficient, very lightweight, generally quite stable as well but cost has been the Achilles heel there. So we look at perovskites single junction have pretty solid efficiency and can be very lightweight and low cost as well. Stability is a challenge. I think tandems are what we
find exciting in that they can kind of check all the boxes doubly in this case. So you can reach very high efficiencies, over 25 percent per module, possibly over 30 in the long run, lightweight so you can get to over two kilowatts a kilogram if you do the right packaging and use perovskite tandems, all perovskite tandems. They can be quite long lived we believe in the future. Still under development though. And ultimately they can help reduce the cost of solar on a per kilowatt hour basis. But the big question for perovskites is always stability. Right? Will they last 25 years? And the short answer is I don't know. I think perovskites have a good chance of achieving
the 25 year lifetimes. And one data point is the experience of silicon, CdTe and CIGS. A lot of PV technologies that have started out unstable and ultimately been able to reach 20 plus year lifetimes. So their history seems to suggest that once technology moves from commercial production into deployment stability problems usually will get solved over time. Maybe that's because of more focused engineering or optimization efforts or more field experience. Obviously this is true only if stability problems aren't caused by some
fundamental unavoidable degradation mechanism which doesn't seem to be the case necessarily for perovskites. So I think our best bet there is to understand where all the holes are and start filling in systematically. And none of this really means that stability ever stops being a concern. But for a lot of other technologies it seems to have become just one of many variables to consider in product design and testing. Whereas for perovskites stability is that one big cold shower that we take in every commercial discussion. So the stability issues aren't clearly
fundamental but they're far from trivial to solve. So I do want to come at this stability question from a few different directions starting with a bit more history. So in the US we've had three major programs for solar RD&D, research development and demonstration before Sun Shot. And this really started in the oil crises years in the '70s.
But the one I want to highlight here is the flat plate solar array program. That was the first major program here in the from '75 to '85. This was very relevant for stability development for silicon. So if you look at this project, this program the goals were really
to build a reliable and standardized silicon module design with relatively high performance for the time, long lifetimes and reasonable cost. And the way this worked was that DOE funded industry silicon R&D and then bought modules. They bought the modules and then ______ researchers at JPO and elsewhere tested them and the test results were used to improve the manufacturing processes so there's a lot of exchange between industry academics, government, nonprofits to really develop this technology and develop manufacturing. And I see a lot of benefits from block buys. It really encouraged the PV industry to use the latest technology. It did evaluate modules using a uniform test or series of tests. And importantly actually created these close collaborations between the manufacturers and the evaluators which led to really fast learning. So in this ten year period we saw
module efficiencies, warrantees and costs improving dramatically. We saw a lot of foundational technologies and manufacturing processes developed for silicon. And I think a lot of industry experts - I wasn't around then - but certainly a lot of people say that FSA was foundational to the terrestrial PV industry. So if you look at where FSA started these are typical solar modules in 1975 silicon modules. And they're actually maybe not that different from the kinds of perovskite modules that you're seeing today. But over that ten year period we saw across these five sort of block buys a quick evolution to the commercial format that we see today for silicon. So the cell packaging factory increased. We saw module efficiency, power and
costs all improve quite dramatically. And probably most importantly it helped make silicon PV reliable. So if you look across this program it helps reduce module failure rates from over 20 percent to 0.1 percent in the last block buy.
So there's a few possible take aways for perovskites. Field testing is obviously critical. We need to move from passing the standard qualification tests to understanding how perovskite specifically fail in the longer term and then design the qualification tests to evaluate those failure modes and design solutions to mitigate them. Obviously perovskites can benefit from a lot of these reliable designs that were developed through FSA and the thin film programs, things like substrates, interconnects and capsulants. And maybe other kind of learning is that government coordination if it's done right can do a lot to push PV technology forward. So perovskite companies and researchers need to work together to advance perovskite reliability. I think it's going to take government and
national lab support to coordinate this kind of program. Maybe a slightly different perspective. This is some nice data from a really great analysis by Dirk Jordan and Sarah Kurtz over at NREL. And this shows that historical, these are mostly silicon module warrantees have often been way out ahead, about five or more years longer than the longest published field tests at the time. So that's interesting. Yeah. To be clear I'm not saying anyone should go and warrantee perovskites to their heart's content today. That would be really bad for the industry. But there's some precedence for
selling PV without fully proving out the warrantee of the lifetime in the field. As long as the science is reasonably well understood. So if we look at how perovskite solar cells might break to try and understand that science there's a lot of different mechanisms so intrinsic and extrinsic mechanisms that we need to understand and solve. So a lot of intrinsic ones which happen even in inert conditions. There's structural instabilities in the perovskite which could lead to decomposition. There's thermal stresses which can cause the organic cations and the perovskites to leave to sublimate and also cause delamination between any of the layers in the stack. Ion diffusion can happen leading to these contact and halide reactions which tend to cause shunts and defects in the cell and reduced performance.
It can also have light induced instabilities anything from halide segregation to dealloying of the perovskite to defect formation. So these are all possible under light which is a problem if you have a solar cell. And certainly there's extrinsic mechanisms like moisture and oxygen ingress which can lead to decomposition as well. So for the intrinsic things you basically just want to turn your perovskite compositions carefully. You want to choose your contact layers carefully and design
internal barrier layers in those cases to try and block that ion motion which is unique to the perovskite. In terms of the extrinsic mechanisms the probably the simplest design strategy is just to choose good packaging solutions. Certainly the better we can do in terms of intrinsically stable perovskites the better off we are from an extrinsic perspective as well. So there's a lot of key stability tests that need to be done. I think light and heat, high
temperatures, high in testing lights is very important, particularly important of perovskites. But certainly things like the MP, UV, thermocycling and combined stresses are all important tests. And it's important to know that you actually need different tests for different applications. So space product has to be radiation hard for example. So at Swift we've been able to pass - just to give you an example we've been able to pass damp heat tests with flexible packaging. So this is just over 1,000 hours the efficiency change for tandems in wide gaps and low gaps. And obviously going for these
flexible applications makes stability challenges more challenging. You can't just use glass as a barrier. But we are making very good progress here. So what's the outlook on stability? I think personally I think it will take a few more years to prove out this ten plus year field performance in reliability. And then you have preventability as well. So it's not going to be a fast process either way. So shorter lifetime applications like these space or UAV, things like that are already in reach today. But if
you really want to go after the longer term applications soon I want to show one possible strategy that you could consider to get perovskites into the market sooner. So the context here is that DOE and others often say that we need 50 year life modules to hit the Sun Shot targets, to hit our climate goals. But we've seen that PV technology just keeps getting better and better consistently and rapidly. Costs are coming down.
Efficiencies are going up. And degradation rates are going down as well. So we're at a point now where the module hardware and the module installation are actually only a small fraction of the total cost of solar and that's especially true in the US. So if this balance of system, these non-module costs dominate then you might think that a long module lifetime maybe isn't as critical because you can take these shorter lived modules and actually replace them one or more times during the system life given that most of that infrastructure is already in place at least in theory. So you can upgrade these modules periodically with better and better modules.
And obviously this is particularly important if you start out with a shorter lifetime. So we did some basic LCOE calculations with NREL benchmark pricing data for US PV systems. This is on the left here for a standard, sorry, standard operating strategy for a PV system. So you incur a bunch of costs up front as you do with a PV system. You'll spend some at the end to decommission. This is for 100 megawatt utility scale system. You can
see in red here the efficiency of the modules degrades over time. And this is within warrantee but it does degrade over time. The degradation rate here is constant because you have the same modules percent per year. The capacity degrades alongside the modules. The EC capacity is - sorry, the DC is usually over size compared to inverters. So that actually stays constant and the generation falls off slightly.
So if you go ahead and include module replacements you see if you imagine one replacement event at year 15 here in the middle you incur module and inverter costs. You incur some installation costs at that time but it's relatively small compared to upfront costs especially with DOS. So at that 15 year mark you get to jump up in efficiency to where you assume modules are at that point. Degradation rate is a lot lower or can be a lot lower. And in this case you jump up your capacity as well both in DC terms and as
you upgrade your inverter or when you replace your inverter you also upgrade so the AC capacity jumps as well. And that's when you get to a step up in your generation. So if we go ahead and compare a few different options for PV technologies so you can start out with commercial technology so this might be a silicon or a thin film, commercial thin film module. You can compare that with a lost cost hypothetical module in green here which has the lower price but also slightly lower efficiency and high degradation rate. And then a third case where you have a high efficiency emerging technology which starts at a higher price, can go to lower, has a very high efficiency going even higher and starts out with a high degradation rate, in this case a ten year lifetime to start and improving over the course of these 30 years. So if you look at how these perform without module replacement you see that in general the emerging PV LCOE tends to be higher by about 15 or 16 percent than the standard commercial PV LCOE for all the system types.
But if you go ahead and replace the modules every 15 years the LCOE for these emerging PV, this levelized cost of electricity it actually becomes highly competitive, right. So its the blue one here compared to the black here. And importantly as you go longer term this grey one assumes that you don't replace but have the ultimate performance for these technologies. You can do better with these emerging technologies than even commercial technologies that are extrapolated out in performance. So the core idea here is to think of modules, PV modules as an upgradable technology and not just this one time infrastructure investment. So by doing that you can make modules that are short lived start out competitive actually with long lived modules. So
again this emerging PV with even a ten year life to start as long as it's improving quickly can have an LCOE that's competitive. And obviously there are a lot of caveats to this. The module replacement concept isn't always economically favorable. So you need to do detailed financial modeling for specific systems. As a general rule you need an efficiency gain over your installed modules to be large enough to justify that extra cost of replacement. And you need lifetime to be improving as well. And we'll probably need module recycling to reduce the environmental
impacts of doubling the number of modules you're using for example. Backward compatibility is going to be an issue where you need to be compatible with the structural or electrical BOS. Otherwise you're not leveraging that existing infrastructure. You might need new interconnection agreements, transmission upgrades to accommodate the higher generation capacity. There's the risk that you get a higher cost of
capital because of using a new technology which might actually negate the LCOE benefit. And certainly there’s not a huge amount of pure economic incentive to do this. You can see the benefit is still not that great compared to just continuing to use silicon. So until you reach that long term end game it's actually not a huge advantage in terms of LCOE. So I think government support for this strategy might be one approach to derisking and scaling up new technologies at a reasonable cost.
But ultimately this concept of module replacement can let PV technologies like perovskite tandems or perovskites in general which could have very high performance but are unproven into the market without that 25 year life. So clearly there are a lot of challenges ahead. I just want to leave you with a different perspective on perovskites and the future of solar. So when I'm feeling particularly nerdy I do like to think of Swift as a nuclear fusion company. They've also raised a lot of money a lot more money than solar so maybe that's a good way to frame it for our investors too. But we like to call this modular remote fusion. Right? And this is actually a
fact. The fun is a free fusion reactor. We do wireless power transfer across 100 million miles which is pretty remote and our modular technology is going to be the lowest cost selector of solar energy. So in short we're making the cheapest fusion reactor in the universe. So I'll actually lean into this a bit. What I think is actually very cool abut light, starlight specifically is that it's the energy carrier of choice in space. Right? That's it. There's no real other option. So if you're going to use this I mean PV is really the best way to
convert energy from this form that the universe likes, electromagnetic waves to a form that humans like, electricity. So what's cool about that is that any technology that we build today to capture the starlight around Earth, perovskite or otherwise. if we make it good enough and efficient enough this technology that we build today can really benefit humanity for all time and that's no matter where in the universe we go. So we've actually done the synchrotron test with our colleagues at Cambridge and found that perovskites are in many ways actually the best technology for Mars and for space. In a lot of early tests they can survive proton eradiation something like five times better than today's leading space technology. They can be a lot lighter weight and they're an order of magnitude lower in cost. So that means if Matt Damon here had perovskite instead of
solar satellite III-V technology or these super heavy panels made out of weird reflective silicon cells, if he had perovskite I think Matt Damon would have had a way better time on Mars. So with that, enough of Matt Damon. Let's wrap up. Swift Solar has a world class technical team, technology in the market that we expect will let us scale up sustainably and profitably. But more importantly I think for everyone out there working on perovskites for other emerging technologies I do think we're on the frontier of an incredible opportunity to build technologies that can serve humanity for a really long time no matter where we end up.
So yeah. Thank you again to the Swift team and our supporters, to all of our collaborators at NREL and Cambridge and elsewhere, to the US- MAP team and to all of the _____ members and to all of you for listening in today. I'm happy to take any questions. >>Laura Schelhas: Thank you Joel. This is really interesting. I definitely appreciate the Matt Damon references at the end. We don’t have a lot of time for questions. But one that has come up in a couple of different forms is related to recyclability and end of life. And I think that this is a really important question given that you're recommending a module replacement strategy. so what are your thoughts on opportunities for
recycling perovskite cells? And do you see a path forward for kind of what the end of life looks like for these? >>Joel Jean: That’s a great question. I think it's going to become particularly important as we deploy a lot of it obviously. So in the early years it's not a huge deal. I think in terms of recyclability we haven't done much work on this. I think there's a lot of interest in it. So there are a couple of points I guess. The actual raw material use in here is relatively small. So that's one small comfort. But assuming as we go and deploy a lot of this stuff it is going to be a concern. So I think there's some early
work that shows that you can pull out a lot of the constituent materials, the lead, the halite, a lot of the raw materials that come out of these modules so you can break it down. So I guess the way we look at it is if you, if there is that demand for it which we expect there will be eventually we can develop recycling programs with partners to actually reclaim modules and break them down. So I think in terms of the environmental impacts of this kind of module replacement, we did a bit of an analysis here looking at life cycle impacts on the environment. So with silicon I mean you certainly have some major impacts and in all cases actually for metal depletion because these different PV technologies all use critical materials, metals. But in
many other categories including carbon emissions and kind of toxicity and all kinds of things from an environmental perspective adding module replacement actually is not so bad. For silicon obviously it increase dramatically but for CdTe and CIGS it has a relatively low life cycle impact and that's going to be similar for perovskites. They are manufactured in a similar way, use very similar module structure. So I think from that perspective replacement isn't going to make a huge difference. Recycling is going to be important either way.
>>Laura Schelhas: Great. Another question here that I have interest so I’m going to be biased and ask it. How much outdoor exposure testing have you conducted so far on your samples or modules? >>Joel Jean: Great question. We have a small test going on I think in Charles living room. But otherwise we have not done much outdoor testing. It’s mostly it’s a very high
priority for us and I think that’s something that NREL and US-MAP can help with a lot. >>Laura Schelhas: Great. I like that answer. And then I think a nice question to kind of wrap up as we approach the hour. In what timeframe do you see solar and renewables completely replacing nonrenewable sources on the grid? What’s your prediction? >>Joel Jean: What’s my prediction? Wow. I think it’s going to be somewhere between 2035 and 2050. Not sure if that’s a good answer.
>>Laura Schelhas: It’s a fun estimate. I think I asked Joe the same question. I think he was a little bit more optimistic. So we can decide on the conditions later. But good to hear. Harrison we’ve just hit the hour. Is there anything you want to
mention? Joe I think you’re coming in. >>Harrison Dreves: Well, no. I was just going to say I would totally defer to Joel since he’s got the business experience and acumen. >>Joel Jean: I would defer to a lot of other people who know a lot more than me. So appreciate that though. >>Laura Schelhas: Going out on a limb. We’ve got people in the chat saying
anywhere between 2030 and 2050. We should take a survey of the community at some point and see who is right. I think it’s fun to think about. >>Harrison Dreves: I think we can make that happen on the webpage maybe. >>Laura Schelhas: Yeah. I think we should do it. >>Harrison Dreves: We could set up a poll if you guys want.
>>Laura Schelhas: Cool. Let’s do it. Guys, anything to add as we approach the end here? >>Harrison Dreves: Just a reminder we will get the recording posted next week on the US-MAP website. So to news and events and then click the link to the webinars page to find that. And stay tuned for our March webinar. We’ll also post that on that page once we get it prepared. Male: Excellent and do check out the US-MAP webpage. We’ll be also posting about
for potential companies interest in staffing needs, the NREL post docs and graduate students are having events that we’ll feature there as well. So I just want to thank Joel again for spending some time with us. I think it was a really, really great presentation. I especially like the power __ I must confess. Yeah. >>Joel Jean: Sounds good. Thank you all.
>>Harrison Dreves: All right. We’ll end it there. Thanks everyone.
2021-02-22 23:08