WINDExchange Offshore Wind Webinar: Technology Below the Water
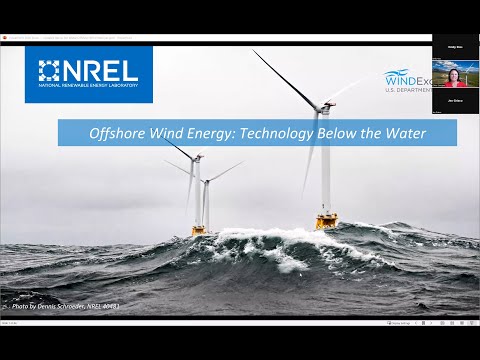
>>Chloe Constant: Hello, everyone. Thank you for joining us today. I'm Chloe Constant, and I am the portfolio manager for Wind Energy Stakeholder Engagement and Outreach at the National Renewable Energy Lab (NREL). On behalf of NREL and the sponsor of today's event, the U.S. Department of Energy's Wind Energy Technologies Office, I want to welcome you to today's webinar.
We're really excited to present today on Offshore Wind Energy: Technology Below the Water. And just to note: this is part of a series of webinars on offshore wind, so if you are interested, I would encourage you to visit the WINDExchange website or NREL's YouTube channel to check out some of the previous webinars, including the most recent, Offshore Wind: Technology Above the Water. Before we begin, I am going to go over a few quick logistics. If we could go to the next slide here.
So we are recording today's webinar and will be posting it to the WINDExchange website and NREL's YouTube channel together with the previous webinars. So we'll let everyone who is registered for this webinar know once those are posted. There's also going to be a Q&A session following the presentations, so please put any questions you have during the presentations in the Q&A function that's going to be at the bottom of your Zoom screen.
And don't worry if there are any questions posted that we can't get to in our time today. We are going to be following up with answers to those in writing, so please do ask away. And if there are any issues with Zoom or with the technology as we go along, we have Emily Klos on, who can help with those, so do reach out to her in case you're experiencing any challenges. But now we'll get to the good stuff. I'm really pleased to be introducing our two great speakers today. If we can switch over to the next slide.
We have Dr. Sanjay Arwade from the University of Massachusetts Amherst, where he is a professor of civil engineering and is the associate director of the Wind Energy Center. He studied structural engineering and mechanics at Princeton and Cornell and works primarily in areas of probabilistic mechanics, offshore wind energy engineering, and reliability. His work has been funded by the U.S. National Science Foundation, BOEM, the Massachusetts
Clean Energy Center, and industry. And we also have Dr. Amy Robertson, who is the offshore wind group manager at NREL, where she leads activities focused on advancing offshore wind design capabilities and practices. This includes leading projects on the verification and validation of offshore wind modeling tools and advancing floating wind control co-design methods and technologies. We think you're really going to enjoy the webinar today. So a big thank you to our speakers.
Thank you also to the project team for organizing this event and WETO for sponsoring. And thank you all again for joining us. Without further ado, I'll go ahead and hand it over to Amy to get us started. >>Amy Robertson: Thank you, Chloe. Can everyone hear me OK, I hope? I'll assume so. OK, so in this presentation today, we're going to cover three different topics, as you can see here on this slide.
First, we're going to give you an introduction to offshore wind energy, and I will be covering that. And then we'll go into understanding seafloor characteristics under part one, and that's what Sanjay will be covering. And then we'll come back to me for part two, which will go over the technology options for offshore wind.
OK, so first of all, why do we want to pursue offshore wind energy? So first of all, for those who are not familiar, offshore wind energy just means putting wind turbines that are traditionally on land, putting them in the offshore bodies of water off the coast of our nation. So why do we want to do this? Well, the first reason is that we actually have the majority of people living in the U.S., especially living on the coastlines of the U.S., are about 80% of the population lives. And so, having offshore wind generation close to where those people live is valuable for having direct transmission without having to drive the transmission very long distances across the U.S.
The wind offshore is actually stronger than it is on land and more consistent, and it's less turbulent than on land because of the fact that there's not a lot of buildings, a lot of obstructions that the wind is flowing over. We're also able to put larger projects and larger turbine sizes offshore, again, due to the fact that we don't have limitations on things like transportation, trying to transport parts over land. We can do those over water, which allows for the turbine size to grow, and we also don't have to worry about getting around obstacles.
So we're able to have the larger turbines and also larger projects, because we have the open space there offshore. There also is a benefit to the economy and workforce for these different regions of the coastline. So we have a lot of ports that have been underused in recent years as the shipping industry has become more and more larger scale, using large shipping vessels, which can't go into these smaller ports that we've used historically. So we actually see a lot of economic benefit to those underused port areas where there's not as much work to be done. So I guess that hits the last point there: revitalizing those ports and domestic manufacturing capabilities that are being underutilized in recent years.
So what is the status of offshore wind globally? Well, as of 2021, the offshore wind projects installed globally amounted to about 50 gigawatts of offshore wind capacity. The average project size is starting to get larger, with some exceeding about 1,000 megawatts, which, to give you some perspective, means it can power up to 1 million homes. And this is actually on the size of other types of generation, like a coal plant or a natural gas plant. So those are also kind of reaching those 1,000-megawatt size. So a wind farm size is very similar to other types of power plants.
Right now, about 99% of the offshore wind turbines that are installed are what we call fixed-bottom designs, where the structure is all the way to the seafloor. These are ones that are installed in shallow water depths less than 50 meters, and we'll be talking more about that—about the fixed bottom designed versus the floating wind designs, which are placed in water depths which are larger. The average turbine rating right now for ultrawind systems is about 10 megawatts, but that's continuing to increase because of cost savings that can be achieved when you grow in size. And so we're seeing about 12–15 megawatts for the projects that are going in around the 2024 range. In general, we are continuing to see overall costs declining, but as we do start to build more systems offshore, we're going to continue to have issues related to the cost of maintaining those systems in the offshore environment. There's a general rule that doing any work offshore is about a 3:1 cost comparison to doing it onshore, so that will be a continuing issue that we need to try to address, including trying to get more reliable structures.
Offshore wind also leverages obviously the land-based wind market but also relies on the existing mature marine industries as well. So, in the U.S. presently, we are a little bit behind the game compared to the rest of especially Europe.
We only have 42 megawatts of operational wind farms in the United States right now. These are two wind farms called Block Island Wind Farm that operates off the coast of Rhode Island, which has a capacity of 30 megawatts and contains five 6-megawatt Haliade wind turbines, and then we also have the Coastal Virginia Offshore Wind Farm, which has two wind turbines at 6 megawatts each, so a capacity of 12 megawatts. These are on two different types of fixed-bottom support structures; one is a monopile, and the other is using a jacket support structure. As you can see in this picture on the right here, while we only have a little bit installed, we have a pathway to installing a much larger number.
So these are showing the different call areas where we're looking to develop offshore wind projects, and you can see that these locations are in all four major bodies of water in the U.S., so off the Atlantic Coast, the Pacific Coast, the Gulf of Mexico, as well as our Great Lakes, where we'll be putting offshore wind farms as well. And to try to drive this offshore wind industry further, the Biden administration has actually set a very aggressive goal of trying to get 30 gigawatts of offshore wind energy into the United States by 2030. So, they're looking to support this by advancing the U.S. wind energy projects to create well-paying unionized jobs, investing in the American infrastructure to strengthen the domestic supply chain, as well as supporting critical research and development and data sharing.
So now, I'd like to move things over to Sanjay, where he'll talk a little bit more about understanding what the seafloor characteristics are. >>Sanjay: Thanks, Amy, and thanks to everyone for the invitation to participate in this seminar. I'll do my best to take you through a little bit of discussion of sort of what soils are in offshore engineering practice, a little bit about integrated site characterization, and then some site investigation and a few specifics on CPT testing. Next slide, please, Amy. Go to the next slide, please. Thank you.
Before I dive into this slide, I'll just mention professors Don DeGroot and Zack Westgate, colleagues of mine here at the University of Massachusetts Amherst in geotechnical engineering, who provided most of the images that I'll be showing today and frankly have taught me a lot about geotechnical engineering over the past 10 or 12 years of our collaboration in offshore wind. You can see here the progression of particle sizes from the smallest clays on the upper left, all the way down to cobbles and boulders on the lower right. I'll talk mostly about clay and sand and then a little bit about cobbles and boulders as well. Each of them have different mechanical properties that have to be considered in design and site characterization. Next slide, please.
So the defining characteristic of clays are that they have this very low hydraulic conductivity, which means that it's very difficult for water to move through the soil, and that results in mechanical properties that are driven by what we call undrained response. Effectively what that means is that the state of stress in the material—for example, due to wave or wind loading on a structure—the state of stress varies faster than the fluid seawater can move through the pores and kind of equilibrate the fluid pressures that are occurring within the soil. This is a very rich field of soil mechanics that I won't go into too many details or more details about today, but I think that's probably the one thing that people should be aware of when thinking about clay behaviors offshore. It's relatively simpler to [distorted audio] of clays from the seafloor and test them in the laboratory.
Next slide, please. Sands, on the other hand, have much larger particles and therefore larger gaps and pores in between the particles. And the fluid is able to move much more rapidly through a sand. Sands therefore display mechanical properties that correspond typically to drained states, which means that the pressures and the fluid seawater can equilibrate more or less on the same timescale as the states of stress and strain in the material are varying.
Sands are very difficult to collect from the seafloor for doing laboratory testing, and therefore much of our characterization has to rely on institute testing. Next slide, please. Cobbles and boulders, these sit at the upper end of our size range, and there are some regions of the Atlantic Coast of the United States where there is some suspicion that the presence of cobbles and boulders could be quite significant.
We're not interested in how cobbles and boulders affect the mechanical properties of the soil as much as whether they provide—may present impediments to installation activities. So you see here a diagram from a public domain report regarding the South Fork Project, and the colored dots in that map on the upper right indicate the presence of cobbles or boulders that may possibly affect, for example, the ability to drive piles into the soil. The image on the lower right is a geophysical investigation, a sonar- or echoseismic result that shows the potential for identifying cobbles and particularly larger boulders with that kind of a site investigation. Next slide, please. The goal in any site characterization is to take an integrated approach, in which a combination of bathymetry, geology, geotechnical characterization, and geophysical characterization are all kind of put together in order to deliver what the foundation and support structure engineers need, which are essentially designed properties for the soils.
These are large teams of people with varied expertise using big and expensive pieces of equipment, including vessels—highly specialized vessels, which can often drive much of the cost of these kinds of site investigations. Next slide, please. Ideally, the outcome of that kind of site investigation is what's called a ground model or an integrated ground model. And the ground model version, of which is shown graphically on the right-hand side of the slide here, is essentially a database of information on structural geology, geomorphology, sedimentology, stratigraphy, geohazards, and geotechnical properties. It can involve software and techniques such as geographic information systems.
There can be iteration involved in developing the ground model, and there can be risk-based analysis of different potential impediments to installation and performance of offshore wind foundations in the offshore environment. Next slide, please. Although I'm an engineer, and professors DeGroot and Westgate are also engineers—geotechnical engineers, in this case, in a sense—site investigation and characterization for design really has to begin with geology. And so I have a couple of quotations here relating to the fundamentals of soil mechanics: "…every soil investigation should include adequate investigation of all geologic features that have bearing on the problem." And then, "…the first step in any subsoil exploration should always be an investigation of the general geologic character of the site. The more clearly the geology of the site is understood, the more efficiently can the program for soil exploration be laid out."
And what I think I'd like to emphasize here is that there is, to a degree, a sequential nature to the geologic and geotechnical explorations. Effectively, the geological explorations, involving things like seismic profiling or sonar profiling, give us a picture of the overall geographic geological structure of the site of interest, the site of potential development for offshore wind. But in general, geological explorations cannot provide soil properties. Geological explorations cannot tell us what the strength of a clay or a sand is in any reasonable fashion. So, while it's useful and in fact necessary to understand the overall geology of the site, such geological explorations must be followed up by detailed and high-quality geotechnical site investigations in order to eventually allow the engineering designers to do the job of designing and then delivering suitable support structures and foundations. Next slide, please.
A word about soil property measurement here. The sort of flowchart in the center indicates that there are two common paths towards the characterization of soil properties. On the left is in situ testing, which in effect means measuring of the soil properties without removing them from the seafloor, deploying probes to evaluate properties, mechanical properties of the soil at and below the seafloor. On the right-hand side of the flowchart is laboratory testing, and of course, that requires removal of samples of soil from the seafloor and then the testing of those materials for the evaluation of mechanical properties in a laboratory—a laboratory, which might be on the site investigation vessel at sea or might be at an onshore facility requiring transport of the samples back to that onshore facility.
In general, there's a tradeoff between in situ testing and laboratory testing. With in situ testing, you have the advantage that the soil is not disturbed. In other words, you're testing the soil in exactly the state and location in which it will be subject to pile driving our foundation construction. However, there are no methods for directly measuring properties, such as sheer strengths of clays, in situ; instead, in situ testing relies on empirical correlations between the kinds of measurements that can be made and the mechanical properties that lead into design. On the other hand, laboratory testing can provide direct evaluation of the key geotechnical mechanical properties, but since it requires removal of samples and transport of samples, it can be very challenging to perform tests on soils that necessarily reflect what the condition of the soils will be at and below the seafloor during actual foundation construction and service.
So those are sort of the tradeoffs between in situ testing and laboratory testing. And most high-quality geotechnical site investigations will rely on a combination of in situ and laboratory. Next slide, please. So a little bit of a summary of offshore wind site investigation methods.
BOEM discusses that these offshore wind areas are frontier wind areas, in which very little geological information exists—in fact, basically nothing below surficial information. There is a range of challenges relative to the offshore oil and gas industry related to length scales and exposures to different kinds of risk and fatigue loading. And then often there's a desire for a staged approach in these geophysical and geotechnical site investigations associated with a balance between cost of exploration and the data that's collected. And one thing that I think is worth noting is that there are different approaches worldwide to geotechnical site investigation and characterization. The Dutch, for example, have publicly funded a lot of site investigational work in their potential offshore wind fields, whereas in the United States, so far at least, we're relying entirely on private-sector-developer-driven site investigations.
Next slide, please. I think this will be my last one, and I just wanted to mention a few details about one of the in situ testing methods that's commonly used: CPT—cone penetrometer testing. In a CPT, effectively what's done is that a small cone-shaped probe is driven vertically downward into the soil column with more or less constant and real-time measurements of things like the end-bearing pressure, the skin friction, and the core water pressure.
You see here vertical traces of the pore pressure, the friction ratio, and the corrected cone resistance. And the gold and blue circles there—or, ovals are meant to indicate the presence of different layers in the soil. So when one transitions for example, from a clay layer to a sand layer, one sees a significant change in, for example, the friction ratio, the cone resistance, or the pore pressure ratio.
These are the kinds of measurements that are made using a CPT test. You'll note that none of them are direct measurements of things like strength, right? So these are not direct measurements of soil mechanical properties. And this is where the correlation comes into play. These CPT measurements need to be correlated to the kinds of mechanical properties—sand friction angles, clay, undrained sheer strengths—that we will use in engineering design. You'll also notice that the data is relatively noisy, and indeed there is a tremendous amount of uncertainty associated with the measurement of geotechnical mechanical properties, which poses some challenges for designers in ensuring that foundations both will be constructible and installable and will provide suitable performance over the lifetime of the structure. I think, Amy, that that's the last one of my slides, and I'll give the floor back to you.
>>Amy: Great. Thanks, Sanjay. So now, I'm going to go into the third component of the presentation, looking a little bit more at the technology options that we have for offshore wind. So here's an overview of what we'll be talking about. First of all, we'll go over the different substructure types, the types of structures we use to support wind turbines offshore, what mooring systems are, and then also talk about the cabling technologies that are used to take the power generated from these offshore wind systems back to shore.
And then finally, we'll talk about decommissioning, which is the removal of these wind turbines after the end of their life. So first of all, here's an overview of some of the different offshore wind support structure types. We have what we call both fixed-bottom offshore designs and floating designs. So again, fixed-bottom are the two on the left here, where you actually have a structure that goes all the way to the seafloor, so you're putting that structure on the seafloor. We have a monopile, which is just a single column, or things like a jacket, which are multimember structures.
But when you get to water depths that are about 50–60 meters deep, it starts to get really costly and unwieldy to continue to build these structures to that depth, and so then we move to what we call floating wind systems, where we now have a support structure that doesn't directly connect to the seafloor but rather floats in the water and is anchored in place using mooring lines and anchors to the seafloor. So you can see three kind of different types here—tension leg platforms, spar, and semisubmersible—which we'll be talking about a bit more in the later slides. OK, so first, some clarification on terminology just to make sure that people are understanding what we're saying when we go through these slide decks. So the support structure for an offshore wind turbine is basically the structure that is used to support the wind turbine, so everything that's part of that structure minus the turbine, so that includes the tower that you'll see for land-based turbines as well, but then the substructure that—the part of the structure that goes below the tower, and then the foundation, which is how it connects to the seafloor.
So that's the general description for the fixed-bottom systems. When you go to floating, you still have the substructure that goes below the tower, but now, again, the foundation is no longer things like piles that are driven into the seafloor, but rather, we're using mooring lines that then connect to the seafloor using anchors. So the foundation is really that anchoring system. OK, so let's talk a little bit first about fixed-bottom offshore wind turbines. So these wind turbines are very similar to what you'll see on land. Some of the differences are: First, they have to be marinized to be able to withstand the ocean environment offshore.
Again, they are fixed to the ocean floor via the support structure, and they have a larger power capacity than is typical for land-based turbines, which we talked about a bit before, because of less constraints for building larger turbines offshore than on land. We also have more complex support structures than what you see on land. On land, you just have that simple cylindrical tower typically. Now, you have the substructure, which can have this multimember jacket or different designs like that.
The offshore wind turbines are also operating in more high-wind conditions, so they're designed to withstand those higher wind speeds and also designed to withstand the wave conditions and storms associated with the offshore condition. For the wind turbine itself, there also may be some differences, because offshore, you want more reliable systems, you want less moving parts. So onshore, you have a geared system typically, but offshore, the systems tend to be more direct-drive-type systems, especially for floating wind systems, where you have a lot more motion. So, this slide shows some of the different types of fixed-bottom design options. We have the monopile, which is just simply continuing that tower supporting the wind turbine into the seafloor and piling that cylinder into the seafloor for attachment. Over 70% of the offshore wind turbines installed to date are those simple monopile structures.
It's obvious… I mean, those are easy to build; it's just a cylinder mainly, so it's a very simple structure and cheap structure to build. But as you start getting to deeper water depths, you'll have to keep increasing that monopile size and that can get to be too costly or too hard to build those larger sizes, so that's when you start to go to things like this jacket support structure, where you have a multimember support system that's then piled down into the seafloor using piles at each of the ends of the legs there. Another option is also gravity-based. Instead of installing these piles down to the seafloor to get it connected, instead you have the structure actually sitting on the seafloor with a wide base. So here, you're just having to get a very flat area, so it does require a lot of prework to get your site kind of nice and flat.
But there, you can then not have to do that piling-type process and just set your wind turbine on the seafloor. A fourth option is a tripod. This is probably the least-seen design out there.
It’s kind of like a jacket structure, but only three numbered legs, and it’s kind of a cross between a monopile and a jacket and offers some advantages but isn’t widely seen at this point. So then floating wind turbines do look very similar to fixed-bottom wind turbines except that we are now sitting on this floating buoyant structure rather than a structure that goes to the seafloor. Because you're not connecting all the way to the seafloor, you're actually getting to be able to be more generic and applicable over more different areas for these floating systems, so you can think about just building one floater that may be able to be used in a wide variety of different areas because the seafloor and the water depth is impactful. Right now, we have, in the U.S., about 58% of our offshore wind resource being in water depths that are what we think are too deep for fixed-bottom design. So, about 60% of our offshore wind resource will require these floating wind technologies.
And at this moment, about 17 gigawatts of floating wind energy has been announced to be installed globally. That’s not installed, but what has been announced to be installed in the near future. But right now we definitely have much smaller numbers of installations for floating wind rather than fixed-bottom, which shows the fact that that market isn’t quite as mature yet in terms of its technology.
So we’re continuing to try to drive down costs from that floating wind systems, and as we do, we’ll see more and more of these being built. So in the U.S. here, we’re showing the four different regions where we’ll be installing offshore wind. And this map tries to show you that breakdown of where we think floating wind systems will be built versus fixed-bottom winds.
And you can see that delineation by the coloring of the light blue versus the dark blue. So the dark blue is simply saying we’re over 60 meters water depth, so we think we’ll have to use floating wind systems. So the interesting thing to point out is if you look at the West Coast, the Pacific region, you can see that basically it’s dominated by that dark blue. So on the West Coast, we really will have to have that floating wind technology used as well as on the North Atlantic area. You can see the delineation of the Great Lakes and the Gulf of Mexico area as well, and in the Great Lakes, there may be a need for pushing wind systems even further offshore because of the people not wanting to see the wind turbines from shore.
So this slide shows some of the different technology options we have for floating wind, and we really characterize these into three different types of systems, and those types of systems are based on how the wind system is being stabilized. So when you think of a wind turbine, this is a very large structure with spinning wind turbine blades and this wind force that’s acting on it as well as these waves acting on the structure. So all of this is contributing, since this is an unconstrained structure, to wanting to tip this whole system over.
So the support structure options are really there to try to figure out how to stabilize the system from not tipping over. And these are actually the approaches that have been used already by the oil and gas industry, and so offshore wind is just kind of adopting those historical approaches. But we are continuing to try to push beyond kind of the traditional designs to try to drive down cost. But for the spar structure, these types of designs seek to stabilize this offshore wind system by essentially placing a lot of mass very low in the water. So as you’re trying to tip over, you have this mass, this counter balancing that overturning moment.
You can see it’s a nice, simple structure, just a simple cylinder as well. But the difficulty with these types of designs comes with the fact that you need a very deep port to be able to build such a deep-drafted structure like this. So what’s more commonly being installed today are what we call semisubmersible designs. So instead of putting a lot of mass way down deep in the water, they have a lot of buoyant structures near the waterline. So as the wind turbine starts to tip, the buoyancy in those structures helps to stabilize the system. The other advantage of this is with the shallow draft, one can build it in a lot more different ports and one can simply tow out the wind turbine from that port already completely built, which requires very little work to be done on-site.
TLPs are perhaps maybe, if you look at it from a technology perspective for just the amount of mass and size of the system, it's kind of the smallest, cheapest type design. It's stabilized by using these tension boring lines that go to the seafloor so that tensioning is creating the stability of that overturning moment. The difficulty of these types of designs is that they require those tension mooring lines for that stability, so trying to tow it out means that you don't have a stable structure.
So you can't just simply tow out a TLP unless there's some type of specialized vessel that helps to stabilize it during the towing process. So we've been talking about the support structures. Obviously, yeah, we need to get these systems out to their location where they need to be installed and then install it to the seafloor. So that process is going to be different for the different types of support structures and foundations that we're looking at. So with monopiles, they're typically prefabricated, lowered to the seabed in a single piece, and then installed with a pile-driven hammer.
So you can think of some of those disadvantages there, because that is creating a lot of noise, a lot of impact to the seafloor that we would like to try to avoid if possible. Jackets and tripods are installed in very similar manners, where we tow it out and pile things into the seafloor. But there are some options, such as suction caissons, where we can kind of improve that process. Gravity bases then are very different in the fact that they're towed to the site, and they're not piled now into the seafloor. Instead, like I mentioned before, you're just resting it on the seafloor, but that requires a lot of preparation of that seafloor area to be able rest that gravity base there.
For floating wind systems, we no longer have to be piling things into the seafloor. We're just having this floating structure. So typically, as we said, especially with the semisubmersibles, we're hoping that we can just build the whole system at a port and then tow that out using just simple tow vessels and then anchor it to the seafloor on location. There's a variety of different ways that process is done. The whole system may be built at the port, or it may be that the turbine is installed later. Since we're still fairly immature in that market, I'd say there's a variety of different approaches still being used for how those wind turbines are installed.
OK, so let's talk a little bit more about the mooring system now. So the mooring system, again, is the lines that are used to connect the support structure for the wind turbine to the seafloor. So if we didn't have these mooring lines, we have the splitting structure, the wind would just push the floating wind system off into the large water depths of the deep, dark ocean. And of course, we don't want that.
We want to keep it one location. So those mooring lines are there to tether the floating wind system to the seabed. The mooring lines keep the system in place, they resist the wind and current forces, and you want to design the system to basically move to the level that you want to design it for. So you can design a floating mooring system to have a very small watt circle, as we call it, or a much larger watt circle, and there may be reasons that you want to have a smaller or larger one.
One of the reasons to have a smaller watt circle is the associated power cable. So we have the mooring lines that are connected to the seafloor, and then we have the cable that’s trying to take the power from the wind turbine back to shore. So that power cable doesn’t like to see a lot of motion; it doesn’t want to be moving and bending. So the mooring system is there to also try to limit the amount of motion that the system sees so the power cable won’t get a lot of motion itself.
And there’s a lot of different designs for mooring systems for offshore wind systems, as you can see here on the right. There’re variations in the configuration as well as the type of materials and how it connects to the seafloor. So the most common ones that are being used today are what we call catenary, kind of slack mooring systems. They rely on keeping the system in place by having a lot of heavy chain-type material on the seafloor itself. The opposite-type approach is, again, the tension-led platforms or taut line systems, which are very vertically placed like this. For these catenary-type systems, we use a lot of chain, heavy chain type material.
For taut mooring systems, they're using things like synthetic ropes, and this is an active area of research, where they're looking at different types of materials that might be used in these mooring systems to provide better stabilization with less cost. And then we have the different types of anchors themselves. So depending on the different type of mooring system you use, you may want to also use a different type of anchor. So you can see a lot of different ones here, from drag embedment anchors, vertical load anchors, to drive plate anchors. The catenary-type systems tend to use these drag embedment anchors that just are very easy to install and not very sophisticated, but it's relying on the fact that there will be no vertical force on those drag embedment anchors.
So that's fine for these catenary systems, where that chain on the seafloor is really making sure that there's no vertical forces on those anchoring systems. But of course, for those TLPs, where you have those taught mooring lines, you do have to be using that anchor to withstand the vertical load, so that's why you're using these different types of anchoring systems. So seabed soil type, as Sanjay was talking about, that will also be a determination of which type of anchoring system you'll want to use.
OK, and then cabling, as I mentioned, that we need to obviously get the power from the offshore wind systems back to shore, so we have two types of cables to do that. The first is array cables, which is the cables that use to take the power from an individual turbine to then a common substation for the wind farm. And then we have export cables, which run from that substation for the wind farm back to land to get it back into the larger grid system. And there's different types of cables that are used for those two different types of cables.
You can see that we use both AC- and DC-type cables. So when we talk about array cables, they're typically just AC-type cables, and you can see here kind of the diameter range and the voltage rating. But when we talk about export cables, we see not just AC- but also DC-types. And the reason we like to go to DC-type cables for export is because those cables are going to be running over very large distances, and for AC, you start to lose power as you travel over long distances, and so DC can be more advantageous. And with these floating wind systems, especially, we may be putting them very, very far offshore, and that export cable may need to be very large to get that power back to shore. There's also work that needs to be done on how we lay out this array cable type system.
And this is really based on how you're also laying out the wind farm. So if you listened to some of these other presentations, you know that there's a lot of work that needs to be done to figure out where to place the different wind turbines in a wind farm so that the shadowing behind one wind turbine isn't affecting the amount of power that another wind turbine can extract. So we want to get them as close together as possible to be able to minimize the amount of cable that we need to place, but at the same time, we need to spread it out so those wind turbines aren't affecting one another. So there's an optimization problem here, obviously, in trying to minimize that cable length while maximizing our power output. And as for placing these cables in this offshore environment, we need to obviously make sure that they're staying safe.
So we first of all make sure that these wind farms are not interacting with a lot of other uses, and that's actually going on in the very early stages when we're planning where we want to put these wind farms. And then these cables are buried to protect them; that's their primary approach for protecting the cables. We obviously need to make sure that that's not too shallow so that there's a risk of damage, but if we put it too deep, that's also going to be expensive and start impacting the environment maybe more. So have to look at the tradeoffs there as well. And there is sometimes where they actually do apply protection to the cables where we can't bury them to make sure that they are protected.
Skipping ahead a little bit here. So, yeah, there's two primary approaches to installing the cables. You can do it where you both simultaneously lay and bury the cables at once, which is nice because it only requires one vessel but can have the operations be slower. Or you can do it in a sequential method where you have two different vessels, one for going and laying the cable in place and then the second one burying it, which could allow for more flexibility.
And in this process, we do need to make sure that we have low-weather environments for these different types of things to be being done. And we also need to monitor those cables after they're installed to make sure that there isn't damage happening to those systems as they mature. As I was mentioning a little bit earlier, the floating wind systems, that array cable will be moving around as the wind turbine moves around, so there's also a lot of work that needs to go into understanding kind of that combined approach to that array cable for floating wind system and the mooring line system so that those two can complement one another. And the way they try to minimize some of this motion is they do use things like these buoyancy modules and bend stiffeners to try to make sure that that power cable isn't moving too much. So this slide just shows kind of an example configuration of what these different mooring systems and cabling might look like for an offshore wind farm so you just get kind of a view of what that might look like. It's not exactly drawn to scale but gives you kind of some insight into what this looks like for a real wind farm.
And then finally, I'd like to talk a little bit about decommissioning. So wind turbines are actually designed to have a design life of about 20–25 years presently. Offshore, we are thinking about maybe looking at longer design lives.
But because of the immaturity of the market, we haven't really met that kind of end-of-life timeline yet for these offshore wind systems, so we don't have a lot of practice yet in what decommissioning looks like. But we can either do what we call full decommissioning, where one has to go and remove that full offshore wind system from the ocean when it's at the end of life, or we can do partial decommissioning, where we leave part of the wind system in the water. And that's going to be dependent upon what the mandates are for that body of water.
In the U.S., the government usually requires that offshore wind energy components be removed down to 15 feet below the seafloor unless they've gotten other types of authorization. But we do have to think about the fact that, as these wind systems have been in the water for all these years, we actually are creating its own kind of marine environment around it. So we are potentially kind of removing a marine environment if we do a full decommissioning at the end of life.
And we need to also think about the fact that that's going to once again disrupt the seafloor and the environment as we do that decommissioning process, just like what happens during the installation process. So to just summarize here, some of the key takeaways I think from this presentation are that, first of all, the technology choices for offshore wind are very dependent on the seabed conditions in a specific area as well as the oceanographic conditions, things such as water depth and the qualities of the seafloor. Offshore wind turbines you can see can be either fixed-bottom or floating, and there's actually different technology options within those.
And for floating, I'd say we're still kind of designing even new types of systems, and we're not kind of in a finalized kind of design approach yet. The mooring lines we discussed a bit. Those are what are used to attach those floating wind turbines to the seabed. And then the cables are the thing that take the power from those wind turbines back to shore.
Those cables are typically buried in the seabed to protect them from getting damage. Both installation and decommissioning of offshore wind projects require consideration of the technical requirements as well as the impact occurring below the water, and these are things that are continuously being studied as well. OK, and then, I think, at this point, Liz, you were going to take over. >>Liz Hartman: Yeah.
Thanks, Amy. So really quick, we're just going to introduce a new webinar series that we have coming up. So with this webinar, we're wrapping up our technical series. So we have a suite of webinars that we'd love for you to check out on other offshore wind technology considerations, and now we're going to be moving into some other considerations around offshore wind, including the development process, economics and workforce, as well as community engagement.
So we'll be reaching out about those in the coming months. And then I think we're going to move into—we've had a bunch of questions come into the chat. Before I dig into the questions for our speakers, there's a lot of questions about if the presentation will be available.
And we will be sending out these slides to everyone who is in attendance here today as well as posting the webinar to the WINDExchange website, and we will send out that URL when we are able to get the presentation up onto the website. So with that… Oh, and one last thing. Apologies. For any of the questions that we don't get to in the Q&A today, we will be sending out answers from different NREL experts after the presentation.
But with that, we do have some questions that have come in for the panelists. So one of the first ones was about different soil types and the LCOE project and if you all have experienced or seen any studies about if different soil types can have a big impact on the cost of the project. I see there for Sanjay already. >>Sanjay: Sure. I mean I think the answer is basically no, that we have good foundation solutions available for the full range of soil types, from soft clays to stiff clays and from dense to sort of medium-dense sands. It's true that different soil types are more suitable to different kinds of foundation installation, but I'm not aware of any strong influence on overall project costs, certainly not that would propagate all the way through to LCOE in a meaningful way.
>>Liz: Great. Thank you. We have another question come in about kind of around the decommissioning piece and if you can reuse a base with a new structure.
So when we're replacing these turbines 20–30 years from now, can you reuse the base of the structure? >>Amy: Yeah, and that's a really great question. I'd say that's another—that's an active area of research that we're looking at. Is it viable? Is it feasible to reuse those support structures and just place a new turbine? Because yeah, the design life of the wind turbine is going to typically be kind of the shortest timeline, whereas the support structure without very little cost can be designed to last many, many more years. So I'd say, yeah, that is definitely a thing that is being looked into, whether we can kind of replace that wind turbine only and keep the support structure kind of at the end of the wind turbine life. I'll also mention that one of the complications has been that, through the years, our wind turbine size has grown and grown, so you will have to consider the fact that a support structure built 20 years ago may not be able to support a wind turbine that's significantly larger, so that needs to be part of the consideration.
>>Liz: Great. Thank you. So there's a question on something we didn't really touch on today, about substations and how many substations are normally needed for a wind farm or per turbine.
>>Amy: Yeah, and I don't know if Sanjay has a better answer. That's something I'm not aware of. I do know that. Yeah, a typical wind farm will probably… Those larger-sized wind farms won't just have one substation; they will have to have multiple substations. But the exact number I'm not sure.
>>Sanjay: Also not something I can really add a substantive response to. >>Liz: Completely fine. No problems there. And we can do a little background research on our end as well. So we had a question come in about the different fixed-bottom structures and when you would, for example, use a jacket versus a monopile. And I think this could go to either of you, either around the geotechnical considerations or just considerations at large.
>>Amy: Yeah, maybe I'll just start from the structural standpoint. I'll let Sanjay say from the soil standpoint. But the structural standpoint, it's typically when you get to the deeper water. So in the very shallow waters, you'll use a monopile. Those are easy to build for shallow waters. As you get into the deeper waters but before you're too deep for floating, that's when you have to go to kind of the jacket-type structures, because you'll have to get a larger and larger monopile, as I mentioned, and it starts to get to be too large of a structure.
So it's much easier to build more like a trust-type structure at those deeper water depths. >>Sanjay: I mean there are some additional considerations involving manufacturability. I mean one of the main reasons we saw Block Island built on jackets had to do with our ability to manufacture those support structures in the United States.
And certainly I saw some comments in the chat about noise related to pile-driving, and there's some thought that the foundation structures for jackets can be installed with lesser environmental impact. So I think everyone should just be aware that there may be some kind of ancillary issues that drive support-structure selection beyond just kind of the best technical solution from a structural engineering point of view. >>Liz: That's really helpful. We had a lot of questions come in around the viability of floating offshore wind and curiosity around what's the commercialization timeline and if you all have a sense of when you would expect to see deployment say on the West Coast.
>>Amy: Yeah, that's a good question. I'll say, commercialization timeline, in general… I mean, we do have floating wind systems out there. Obviously we don't have a lot yet, but there is plans for a lot of floating wind, I'd say, in the very near future. We are starting to get to the kind of commercial wind farm scale at this point, starting to get close to that.
In terms of directly in the U.S. and the West Coast, yeah, it's not directly in my head. We're thinking kind of in the 2030 timeframe, but don't hold me to that. Maybe we should check that.
I don't know if Sanjay has a better answer. But it's not something that's just fresh in my mind. >>Sanjay: I’m going to stay away from trying to predict the future here. >>Amy: [Laughs] To not get in trouble. But it's not immediate, but I would say it's not way, way far off.
>>Liz: Good answer. Good answer. So I'm going to ask one more question, and then we'll let everyone go.
We went into quite a bit of detail around cabling, and there was a question after those slides about is there a standard depth for cables to be buried, or is it really dependent on where you are? >>Amy: So yeah, I'll have to punt on that one, unless Sanjay has an answer. Yeah, the cable standard is not something that I'm familiar with. >>Sanjay: Likewise.
>>Liz: All right, well, we will follow up. We've got a lot of folks here at NREL who are thinking about this stuff. That's one of the things we do after these webinars is send responses to the questions that came in. So with that, thank you to Sanjay and Amy for being willing to come to talk to us for an hour today. Super informative.
I know I learned a lot. And watch out for NREL's upcoming webinar series. We will be reaching out.
2022-07-29 03:06