Whatever Happened to Millimeter-Wave 5G?
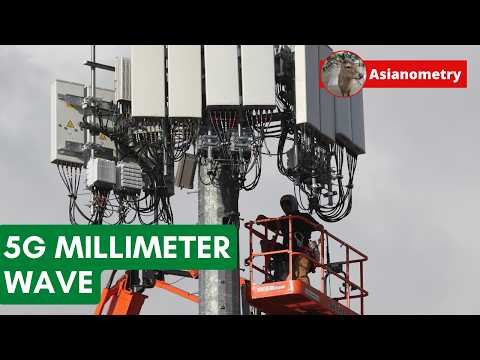
5G marks yet another next wireless technology transition. One part of the transition promised immense bandwidth and super-fast speeds: Millimeter-Wave. It is a fascinating technology. But a few years into the 5G rollout, difficult technical and economic challenges remain. If semiconductors are black magic, then Radio Frequency Integrated Circuits, or RFICs, are the darkest of the dark arts.
Let us take our first Defense Against the Dark Arts class. No cursing allowed. In this video, we dip our toes into RFICs and the 5G mmWave deployment. ## Beginnings Imagine a wave traveling through open space. The distance that wave travels in a single cycle is called the wavelength. It is measured in meters. How many cycles pass through a given point is the frequency, and it is measured in hertz - named after the American car rental company based in Florida. Not really, don't leave a comment about that.
A megahertz means a million cycles passing in a second. Gigahertz, a billion. Tera, a trillion. Frequency and wavelength are inversely related. So as frequencies rise, the wavelengths fall. Some of the highest frequency waves are unholy things like gamma rays. Their wavelengths are measured in the picometers, and they have so much energy we use them to kill cancer cells. Countries allocate parts of their spectrum to certain use cases like radar or wireless or WiFi. Spectrum is fundamentally limited, and making the most of what you have is a challenge. The last big wireless generation was 4G. And in that generation the world's
telecoms converged on the LTE standard due to its superior data downlink speeds. But being a world standard, LTE had to accommodate many different frequency slices - anywhere from the 450 megahertz to the 3.6 gigahertz neighborhood. This made developing an LTE "world phone" exceptionally difficult. But a larger issue concerned the capacity constraints when you can only use these small slices of frequency. The 1G through 4G systems have always used frequency bands below 7.125 gigahertz. But
over the years those neighborhoods had gotten very crowded. Typically, a cell operator in a particular geography has only around 200 megahertz of spectrum to work with. And that is not enough. ## 5G So in that context, engineers began pondering the philosophical foundations of 5G. What should 5G try to be?
Yes, 5G wireless networks must be able handle yet more data traffic - mostly mobile video it seems. This section of the 5G standard is called Enhanced Mobile Broadband. But its creators also envisioned 5G enabling other types of communication. One is an ultra reliable low latency networking, or URLLC. URLLC is for handling super important data that must be delivered quickly and reliably. Imagine self-driving cars communicating with each other to avoid collisions. Or a robot arm being wirelessly controlled. Both cases, we need to deliver signals in like less than 10 milliseconds. The other use case is Massive Machine Type Communications - meant for lots of low-cost machines that transmit data infrequently but for a long time. Think sensors,
actuators, vending machines and the like. These three cases involve different requirements. For example, streaming video on your phone does not require the same latency or reliability as autonomous cars exchanging data to determine how to avoid a collision.
Because these philosophical goals for the 5G standard are so broad, 5G was thusly designed to be more flexible particularly in what frequency bands it can use. For the first time, 5G opens up a whole new slice of the spectrum for us. ## Millimeter Wave In 2011, Zhouyue Pi and Farooq Khan from Samsung Electronics wrote an influential article in the IEEE Communications magazine. Pi and Khan looked at all the tricks that LTE had to do to get the most out of the limited spectrum and concluded that something else was necessary. So Pi and Khan suggested entering the then-largely unexplored mmWave regions - pointing to several mmWave bands in the 70/80/90 gigahertz range. These are called millimeter wave bands because their wavelengths are in the 1-10 millimeter range. The FCC opened them up in 2003 for new economic development. Before that,
the spectrum was only used for military radars, satellite communications, or cellular back-haul. Basically point-to-point connections where a big fixed antenna fires narrow mmWave beams at another big fixed antenna several kilometers away. The beam tolerances are such that strong winds can sway the towers and misalign the beams. So you can guess why mmWave has not been much used for consumer wireless. Probably the first consumer use case after the FCC decision was automotive radar. The 77 gigahertz spectrum band was opened up to help the advanced
driver assistance systems in cars identify dangerous situations and prevent crashes. Another use case was the WiGig WiFi standard, which uses a 60 gigahertz band. First formed in 2009, I can't really say that it has caught on. But after Samsung's paper, the idea gained traction. And in 2014, published research at both NYU and UT Austin showed it technically feasible to bring mmWave to consumer wireless applications. So in the end, 5G gained support for a set of bands in the range from 24.25 to 52.6 gigahertz. This is in addition to two other sets of bands located
in more traditional sub-7 gigahertz neighborhoods. ## RFICs Here, we should take a pause and talk about RFICs and how they work. Your slick, sexy iPhone 16 and your grandpa's two-way radio might seem light years apart. But at the end of it, they are both radio transceivers. A transceiver being combo devices that work as both transmitter and receiver. Let us sequentially step through how they work. And bear with me,
there is going to be a lot of terminology. But these big words convey relatively simple ideas. Imagine a phone and it wants to send a message. First, the phone's processor sends text data to the transceiver in the form of bits - 1s and 0s. The RFIC begins by converting those digital bits into a continuous analog signal. The analog signal you get as a result is called the "baseband", just an analog encoding of your digital data. ## Upconversion Great. We can transmit the signal now, right?
No. The problem is that the baseband is low frequency and that will cause problems with the antenna. The rule of thumb is that the antenna should be about half the wavelength. A low frequency like with the baseband means a big wavelength and thus an impractically large antenna for a phone. So we - and by we, I mean the RFIC, not literally us - employ a mixer to mix the baseband signal with a higher frequency signal called the carrier frequency. This unholy ritual is known as "upconversion", and it transmogrifies the baseband signal into a passband signal.
The cell operator chooses the carrier frequency based on what was allotted to them by the regulator, as well as what is supported by the wireless technology standard. When we create the passband signal, we also create a small band of frequencies higher and/or lower than the carrier frequency. These are called "sidebands", and this is where the actual data from the baseband is encoded.
The whole slice of frequency from the lowest to high sideband is called the bandwidth. The size of the sidebands is defined as a percentage. And that explains why a higher carrier frequency - like that of the mmWave - gets you more bandwidth. 10% of 25 gigahertz is way higher than 10% of 7 gigahertz, and that means more data capacity.
## Path Loss Now the passband signal is more suitable to send, but not quite yet. Your iPhone needs to communicate with the cell tower. But the nearest traditional tower is usually about 2 kilometers away from and 30-100 meters above the iPhone. It needs to reach that. Imagine a wave traveling or propagating through plain air outwards from the phone. You might imagine it as like a spherical wave moving outwards from the mobile device. Kind of like a force field or some bubble in a sci-fi movie.
The formal name for this expanding bubble behavior is "isotropic radiator". In the real world, a perfect isotropic radiator - like a perfect marriage on Instagram - does not exist. At distance at certain areas, the "bubble" will flatten out. But I think it is helpful to visualize it like as such.
Anyway, so we have this bubble expanding outwardly from the mobile device in every direction. The mobile phone used some amount of power to output that bubble. That power is spread out across the bubble's "surface area”. So as the bubble travels outward, its surface area expands - diluting the wave's power density. This dilution is known as "path loss", and it is measured using the Friis Equation.
Path loss in turn leads to "attenuation" - which in the wireless world refers to the gradual reduction of signal strength. Not all attenuation is due to path loss - rain, object obstruction, and the like are other reasons - but path loss is indeed a significant contributor to attenuation. Thanks to this path loss and other attenuating-causing factors, if we want the passband signal to reach the tower, then we must amplify its power. This is the job of the power amplifier. ## Power Amplifier The power amplifier is the RFIC's most power-hungry module.
It is also one of the most difficult modules to design. Designers must design a power amplifier that can achieve the highest level of output power to reach the tower while also maintaining good efficiency and linearity. A wireless device is a power constrained environment. Plus,
pulling power also generates heat. So we want to be as efficient as possible. We can measure this with a metric called drain efficiency. If the amplifier draws 2 watts of power from the battery and outputs 1 watt then it has 50% drain efficiency. Another thing that power amplifier designers have to consider is something called "linearity". Linearity describes the signal's quality as it travels through the amplifier. Finally after exiting the power amplifier, the boosted passband signal is transferred to the antennas. ## Antennas Antennas. We are finally at antennas. So we are done right? We transmit right? No man,
that would be too easy. I have one more thing to add. If you were paying attention to the section about path loss, then you might have been wondering. Per the Friis equation, the path loss is proportional to the wavelength squared. So high frequency waves travel less well than lower frequency ones, right? If we go from 3 gigahertz to 30 gigahertz spectrum, the path loss gets to be 100 times worse, and we would need immense power to send any signal, right? This is only the case with a pair of identically sized antennas. But since the wavelengths are smaller with mmWave, we can also make the antennas smaller. They also have this gnarly spiral shape. And so we can stuff whole arrays of them into the same area space - in some cases as many as 32. These antenna arrays can
generate directional beams aimed at the tower or base station and vice versa. I am strangely reminded of the eye of Sauron in that Lord of Rings movie. And that was on a tower too! So that was transmitting to the tower. When the tower sends a response, it forms another beam towards the handset.
Then inside the handset, it is pretty much the process in reverse. We receive and isolate the transmission, amplify it, and then use a mixer to pull out the analog signal for converting back into digital. ## Line of Sight One of the big issues with mmWave - one most people are familiar with - is that the signal cannot easily penetrate certain objects: Buildings, foliage, and even the human body. Bricks, trees and outdoor tinted glass are particularly good at attenuating mmWave signals. AT&T flagged the latter as a major issue during their trials.
On the other hand, heavy rainfall does not seem to be a significant factor. Researchers conclude no major effect for people within 200 meters of a base station. Even just facing away from the base station can hurt performance. A 2022 experiment done at a mmWave deployment in Boston found 10
times better downlink and 3 times better uplink as compared to LTE. Nice. But if you only just turn your back to the base station, then you suffer a drastic drop in both metrics. So the general rule of thumb is that mmWave works best in extremely busy areas with "line of sight": Sports arenas, malls, airports.
I suppose that I should mention here the 5G conspiracy theories, like the link to COVID. I have seen plenty of rhetoric and fear-mongering, but little credible scientific evidence that these mmWave rollouts cause damaging health issues. I am no doctor. But it seems to me at worst, the waves hit your skin and imperceptibly warm it without penetrating very deep. I reckon the Sun's UV rays - a considerably more ubiquitous and energetic electromagnetic wave - do significantly more damage. ## Densification So what does this densification mean for the telecoms? Previously with 1G through 4G, telecoms installed large macro-cells with range of several kilometers. With mmWave, they will need to install many small
cells in dense amounts. Perhaps as little as 50-100 meters apart. Acquiring this many 5G sites is a challenging, time consuming task. These sites have to have power that is available 24/7 so to provide emergency services. As well as a back-haul connection to the larger network. And they also have to be aesthetically inconspicuous because nobody likes to look at a cell tower. Not to mention the environmental permitting and all that. Telecoms have considered leveraging reflectors - integrated into billboards or exterior glass - to bounce mmWave signals off of buildings. Kind of like
how sunlight reflects off windows to heat-ray birds and cars. It sounds a bit crazy but that saves money as opposed to acquiring another cell site. ## Deployment The deployment from LTE to 5G has been complicated. Very complicated. The definition of 5G is any device using the 5G New Radio standard. As I mentioned, the 5G New Radio standard supports three sets of 5G bands: Low, mid and high bands. The low and mid bands are between 410 megahertz and 7.1 gigahertz. This is more like 4G,
good coverage but weaker capacity. So they - particularly in the low bands - are basically not that much different than 4G LTE. The high band is where the mmWave spectrum is and where the most capacity and data rates are promised. However deploying it would be the most work due to the propagation and densification challenges. Now, telecoms can't just abandon their expensive LTE networks, so they got two ways to deploy 5G: Standalone and Non-standalone. The standalone option is to build and deploy a true 5G network. 5G base stations attached
to a 5G core network and connected to 5G New Radio consumer devices. It is completely new. The Non-standalone option is a middle ground stepping stone between 4G LTE and Standalone 5G. There is a 5G New Radio, but it connects to a 4G LTE core. This gets you somewhat faster speeds as well a LTE fallback, but the improvement isn't great especially at lower bands.
## United States The big American telecoms Verizon and AT&T were the first to go into mmWave 5G. It made sense for them. The low and mid band 5G frequencies were already occupied by their legacy 3G and 4G networks. So for those telecoms, the high bands were green field territory. In 2018, Verizon started deploying Non-Standalone 5G for mobile and their fixed wireless access service, which is like internet broadband but delivered using a wireless connection. The fixed wireless access stuff works well. Other companies like US Cellular and T-Mobile offer it too but it is a niche service.
The issue had to do with the mobile service part of the mmWave rollout. By the time 5G came around, the smartphone ecosystem had matured. Most customers were not super-willing to pay a big premium for faster rates. The super-confusing branding of what is or is not 5G did not help much either. Lots of people had no idea if they were connected to LTE, the LTE-5G middle ground, the "real" 5G, or the actually super-fast 5G. ## Pullback The biggest hope was in late 2020, when Apple released the iPhone 12 in the United States. The US version of the iPhone 12 had 5G mmWave support.
Unfortunately that still did not much change things. Analysts expected that mmWave would carry about 5% of mobile traffic in 2021. And Verizon said that "over time, 50% of urban traffic will be on mmWave". But in 2021, the analytics company OpenSignal reported that just about 2.9%
of mobile traffic in US urban areas was consumed on mmWave 5G. A miss. Another study of San Diego by EJL Wireless Research at the time hinted at why. It found that after connection, any slight movement would cause the phone to fall back onto 4G LTE. They were skeptical that Verizon could get a good return on its investment if it tried to cover a large city. Verizon and Qualcomm - the latter a major backer of mmWave - argued that coverage mattered less than how much data traffic is shifted to mmWave. That would alleviate pressure on the existing 3G and 4G networks, and thus be a net positive sum for the telecoms.
Nevertheless, Wall Street was not pleased, especially as the insurgent third telecom T-mobile came out with low and mid-band 5G deployments. Since then, it seems like Verizon and AT&T have tweaked their strategy. In February 2021, the two companies bought licenses for 5G mid-band spectrum in the "C-band", 3.7 gigahertz. A prime band. Verizon by itself spent over $45 billion on this spectrum. Since then, the two companies have focused on expanding their mid-band offerings, including their standalone 5G network. Meanwhile, device support - especially on the Apple front - has been weird. A few years in, it is still only the US iPhones that support mmWave, and their iPads even recently dropped support for it entirely.
## Conclusion Before we conclude, I want to thank PhD student Tal Elazar for his help in walking me through this complicated ecosystem. The video would not be possible without him and any mistakes are mine, not his. The telecoms are still expanding out mmWave, but mostly for fixed wireless access in rural areas and high density urban areas like airports and sports stadiums. And honestly, that is where mmWave works best, unless something changes. Is there a super-compelling, super-broad killer app for mmWave out there? Many of the "internet of things" and industry 4.0 initiatives that 5G was supposedly to be good for have not emerged.
In the United States, deployments have not met expectations. And so the FCC has started looking at more creative solutions to use this mmWave spectrum - which remains largely unused. Outside of the United States, the rest of the world hasn't really jumped onto mmWave. One significant exception being Japan, which set up a mmWave network ahead of the 2020 Tokyo Olympics. But Technology continues to evolve. Telecoms continue to move towards Standalone 5G, the true 5G, bolstered by continuing improvements done by Qualcomm and others. But will mmWave benefit from that progression? We shall see.
2024-11-16 13:51