Turning the Sun Into a Giant Telescope with Dr. Slava Turyshev
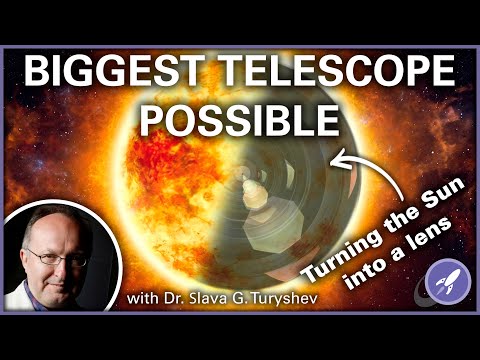
Fraser Cain: Hi, everyone, I'm Fraser Cain. I'm the publisher of Universe Today I've been a space and astronomy journalist for over 20 years. And with these interviews, I try to bring you behind the scenes so you can hear the space and astronomy research the ideas that are coming directly from the scientists themselves. And today, I'm joined by Dr. Slava Turyshev, Dr. Turyshev. Who are you? What do you do? Dr. Slava Turyshev: Fraser, very nice to be here. Thank you very much for inviting me for the conversation here of what I'm doing. What am I doing? I am an astrophysicist at NASA Jet
Propulsion Laboratory. And I'm interested in studying wherever gravity is playing a significant role in a phenomena where gravitation relativistic gravitation is a part of life. This is what I'm interested in gravitational waves study in general relativity in the in the Solar System, looking at anything, which is anomalous in the motion of spacecraft and the Solar System looking for anything that would resemble the next, the next theory of gravity, because we understand the general relativity may not be the final theory of gravity.
So anything that relates to that is excited me. And so there are other things I'm doing looking for asteroids that are probably, you know, the Earth's crust and orbits, there may be some minutes at some point, some of them will come close to our planet. But that's something is not that we that we will be talking today about my main interest these days is solar gravitational lensing. Why? Because we are going through a very significant period in the history of astronomy, because for the last 20 years, we witness and a significant number of exoplanets discovered by modern techniques. And so we use telescopes on the ground, we use telescopes in space we use, we built more telescopes, and we will be looking for more exoplanets orbiting nearby stars. So but the challenge here
is that we will never be able to see them those planets directly. Because our classical telescopes are not allowed to do not have evolved will not allow us to do that, because they're magnifying power is very limited, their resolution is very limited. So even the nearby planet, like Proxima b, we will not be able to see the surfaces of that of that object. And with a solar gravitational lensing, we at least have a good promise that, at some point, humanity will be able to see surfaces of exoplanets all the way to 100 light years away. Fraser Cain: So let's talk about this idea of just like a gravitational lens. First, what is it?
Dr. Slava Turyshev: When light travels in the vicinity of massive body, the light trajectory is altered, it's not moving in the straight line anymore, the trajectory is bent towards the body. And essentially, if you take two rays that are going on opposite sides of that, a massive body, at some point, the both race or the bent will be bent towards the body, and then at some point, they will intersect. And
so they will come into focus. And so but now imagine that the body is spherical, so you have many, many more rays go in and around the circumference of that spherical body. And essentially, when the rays intersect, there will the gravity sort of like the gravity of that massive body acts as a lens. And so similarly to optical lens lenses that we have, gravity acts as a lens that focuses light, it has a very interesting aberrations like spherical aberration, but with the sole gravity that we have, in the Solar System, are the most massive bodies of course, our is the Sun. So the Earth the Earth are also Jupiter
may also be acting as a lenses, but then this lens is extremely weak. Sun is the most massive body in the Solar System. So but when we use the Sun as villains, we suddenly have a gift from nature. Why, because in the focal area of the solar gravitational lens, the light is amplified by a factor of 10 to the 11th. It's 100 million times. And now we're talking about not only significant light amplification, but also M lower resolution. Because we know that from classical diffraction
theory, we know that our lambda over D, well, wavelength and the diameter of your telescope gives you a resolution of your optical system. In the case of the Sun, the D is the diameter of the Sun. So we're talking about nano point one nano arc seconds, which is not available by any other techniques. We just don't have technologies to do that.
Fraser Cain: So can you give us some perspective, like if we were some super advanced civilization, we were able to build just a really big telescope. But how Hubble style or James Webb style how big To give a telescope we need to build to match the magnification potential of the Sun as a gravitational lens, Dr. Slava Turyshev: I will answer this in a different way. Let's take our own planet Earth, the diameter of that planet is about 13,000 kilometers removed planet at 100 light years away from us 100 light years it's a reasonable distance. So to image that object with just one pixel using diffraction limited telescope, you need to have a telescopic aperture of 90 kilometres. It's nine zero kilometres, right? It's just one pixel, one pixel. But we all with our cell phones, with our
proliferation of imaging technologists, we used to have megapixel images just give you a megapixel image of our planet. That's if you have like blue with a pale blue dot that Carl Sagan named the picture that that taken off our planet, it's not enough, we don't, we're not happy with just one pixel of our pale blue.we want to have multi pixel resolution of those objects. And so that if you want to have 100 pixels by 100 pixels
across, you need to multiply that 90 Kilometer aperture by that number. If you want to have megapixel images, you need to multiply that 90 Kilometer aperture by 1000 times. So that's not possible Fraser Cain: to a 90,000 Kilometer telescope, single lens telescope to give you both the angular resolution and the increase in brightness. Absolutely, that's, that's a
very big telescope. Dr. Slava Turyshev: It's something that we call tyranny of diffraction limit. Because to be able to restart resolving
objects, and meaning you can separate details on the surface of our of distant objects, you need to have larger aperture. This is why astronomers always wanted to have a larger aperture. And so but in the case of distant exoplanet, they're too distant and led to faint. So when you image exoplanets, we
are dealing with two problems. First problem is that there are things that are not self luminous. So you're the only reflect light, and they emit infrared. So they reflect light and optical from their whole star. And then they emit their own light infrared. So this is the typical spectrum that we
have to observe. And they are extremely faint. So the typical objects that we will be looking at there in magnitudes, maybe 3759, magnitude will have absorbed anything like that, Fraser Cain: right? Like, we had like what into the high teens like the exactly, the team. Dr. Slava Turyshev: Yeah, magnitude is pretty much a
limit, or for many for many instruments for many wishes. But we're talking about four to seven magnitude, it's not possible with the best techniques that we have. So it's, they're faint, but also the extremely distant, right.
And so to deal with, with that, we need to have a large amplification factor to that amplification comes usually with a larger collection aperture. So the large aperture you have, the more amplification you get, Fraser Cain: right? So if we use say interferometry, we could build, say, put to Hubble Space Telescope, separate them by 90,000 kilometers, we could get the resolution, but we wouldn't get the amplification that would be that we would need to be able to see the so we had to quasars really close together. They're both very bright, and we could see them. But, but we wouldn't be able to see faint objects like another planet Earth, purely looks at interferometry Dr. Slava Turyshev: with interferometers. It's another interesting story because of what we will what we would get with interferometers. And of course, of course resolution, the larger the baseline, the better the higher the resolution of the interferometer. But we but if you want to use
interferometer for imaging, so then we need to have variable baselines and those baselines would have to rotate. And to cover something called uv plane, we need to have separate pretty much there is a field aperture. So we need to fill the temperature with different baselines means that that interferometer needs to change our baseline. So let's say from one meter to 90,000 kilometres, and then it needs to change our orientation. So it's not only in one linear configuration, it has
to rotate in a sense. And so this way you can think about trying to reconstruct a field aperture instrument, but you need to do this fast because planet rotates everything moves. So with interferometry, you get resolution only in one dimension, but it's not it's not the whole picture but interferometers usually you expect that interferometer let's say you have 10 meter telescope, two meter telescopes separated by 90 kilometers. So 10 meter telescope is a big enough, but you need to be able to block out the light from the whole star because it the field of view of the telescope is large. So it will pick up a light from the whole star. So you need to block
that light from the whole star, you need to have a chronograph and usually internal chronograph will not work you need to have starshade. Right? So imagine now interferometry, you have to start shades that are moving in unison with this two telescopes. Right? Right. But it's not, it's not all, because when we look at those objects, they usually move in the inner part of their whole star system, meaning that there will be a lot of zodiacal light. So zodiacal, to deal with zodiacal to be able to build in signal to noise ratio seven, you need to integrate at least 4000 years to get the Sun are several may be familiar in yours for some systems. So interferometer will give you some sort of hopeful resolution, but it completely impractical, because ultimately, you will not be able to see the restaurant planets may be seen directly, you know, like Jupiter super Jupiter's their presence in orbit and the Hotstar. Right, but to be able
to resolve even the Earth orbiting around the Sun from the distance of let's say, 10 per second, maybe 30 per sec. It's not possible within the realistic mission lifetime, we're talking about 1000s and 1000s years. Fraser Cain: All right. All right. So you have you have dashed our hopes for how powerful orbital telescopes are going to get in the reasonable future. So then let's talk about the power of the gravitational lens using the Sun as the gravitational lens. How does that compare to that 90,000 Kilometer telescope that we talked about earlier, Dr. Slava Turyshev: if I take a one meter telescope, and move it
to the focal region of the solar gravitational lens, I can use that one meter telescope and build an image of Proxima b within probably three months. So meaning that the Proxima b if I if I take our own Earth, and Proxima b will be, let's say, the Earth will be orbiting Proxima Centauri system. And so I can make that image with megapixel resolution within a month Fraser Cain: mega pixel mega pixel mega pixel. So that would
be about a million pixels to 1000. And aside, you would have like that's as good as a old digital camera picture. Dr. Slava Turyshev: And that's where this SGL comes. The promise of SGL is that we can have megapixel images of especially nearby planets, let's say up to 10 to 15 per sec. So we should be able to get maybe not megapixel, but maybe you know, killer pixel images, let's say maybe 400 by 400, maybe 600 by 600 pixels. And so with this weekend, we are talking about resolution on the surface of roughly maybe 25 kilometers on the surface of those subjects. And so now we're talking about
continuing the lines, weather patterns, topography, icecaps, the structures, but not only that, with SGL, we're talking about spectroscopy as well. So with spectroscopy, you can actually in the visible years, there are some, some weak lines of water, methane, oxygen. But then if you go to infrared, infrared is amazing. Because with infrared, you can meet infrared, you can actually detect a lot of interest in lines with SGL. And so now we're talking about looking at the surface spectroscopy, it's not atmospheric spectroscopy, it's surface spectroscopy. So because we will be able to compensate for the overall rotation, and so remove the cloud cover and literally peek under the under the clouds and start looking at the topography of that of that object. And so that's what SGL
gives you with, we are not even, I mean, most of the exoplanet community are focusing on the efforts of addressing what science can be derived from unresolved imaging of a single target. So we are not we are not resolving that object. We just sort of broadband spectroscopy and imaging of that sort of diffraction limited pixel. And so realistically, no imaging science actually is discussed within exoplanetary community spectroscopy broadband spectroscopy for global global sort of spectroscopy of planetary scale. Now, with SGL, you can actually do a surface correlated spectroscopy that means if there is a swamp on the planet, we know that there is a swamp because methane is being emitted from that part. If there is some transition from ocean to continent, we know where this transition is happening because we will be able To detect water detected in and detect other elements from from the continents. So that's type of science resolved spectroscopy and sort of surface spectroscopy and that's something that has not really been addressed by any other missions. But with the
with SGL we can do that. Fraser Cain: Now, what is the region now you talked about the focus region from the Sun as gravitational lens, how far away is it from the Sun? Dr. Slava Turyshev: Absolutely, this is because the gravity is extremely weak. And so the focusing of, of light by solar gravitational lens is the focal region begins at 548, astronomical units away from the Sun, opposite from the from the target, we need to move in opposite direction from the target. Basically opposite from the on on opposite direction from the Sun. And so the distance is 548 astronomical
units away. Right. So what the challenge our challenge resistance, so our there is a great promise, but the challenge is the distance to get there in reasonable timeframe, 25 years, because that's what we will LightSail Icarus LightSail is a planetary society mission that is now orbiting Earth, Icarus is Japanese jugs of warm admission, going all the way to Venus essential using for solid momentum. And soon with the launch of SLS, we will fly another mission built by NASA and the Marshall Space Flight Center. So So resealing is now getting into into very interesting part of development because two technologies are coming together, first of all, a small spacecraft. Small SATs similar to those that were flown
on InSight lander on Mars to Marco's to Marco CubeSats. So, those spacecraft are now very capable there, they can benefit from ongoing revolution into electronic world. So all the technologies all the instruments are now miniaturized. Putting this together with the solar sails, now you have a viable mission. And so viable mission are you need to do what you need to unfold your sail. And so then you are using the sail as like as a sailboat essentially, on any sailboat has what has sail and has rudder, and you have you have solar wind, essentially you can go against the wind pretty much using us and using the wind on the sailboat with the solar sails what you have you have sail, you have solar radiation pressure, and you have reaction wheels on board reaction wheels that allow you to actually choose a course such that you will be dumping your kinetic energy and the fallen onto the Sun with pretty much a full month after launch. With the C three zero you're actually from
Earth high Earth orbit, we are flying by solar perihelion, we open the sail, and we now picking up a lot of solar radiation pressure. The closer we come to the Sun, the more the higher the solar radiation pressure will become. With the technologies that you have today, we use in sails, the developmental in the laboratory today, we can fly as close as maybe point to astronomical unit from the Sun. So and that allows us to pick up velocities of roughly three times the Voyager velocity. Voyager is going with about three astronomical units per year. That's the velocity of wager in New Horizons with the
current currently available technology for solar sail and we can go seven to nine au per year. And so 79 the European region with the technology we have today, we can travel travels the Solar System much faster. So but then a sail sail technologies that we're developing, for example, a group led by Professor Arthur DuBois in UCLA builds new sail materials that will allow us to get to almost a 15 solar radii and not being burned by the solar hit. Essentially it's very reflective material very lightweight. So coming that close you can think about reaching the velocities of all the way to pretty much three astronomical units per year. So
to get to solar gravitational lens, we need to have about 25 au per year with this velocity we will go we will fly by the Sun will be good at velocity and reach the solar gravitational lens focal region about 25 years long time long, long wait, but definitely worth Yeah, so this is our main challenge. People usually say Slava you need to move faster or live longer to reach the solar gravitational focal region. I said, I usually say I prefer to do both. I want to move fast and live longer. So
in the in this case, by the time when I'm 100 years old, everyone sees that surface of exoplanet that will be imaged by a mission that we will launch and registered to Fraser Cain: well hopefully You'd be able to get a spacecraft named after you, like Parker, in and be alive to see it to see launch. Now, I mean, there are technologies that could take you faster, I mean, so I see you're sort of doing an Oberth maneuver past the Sun, getting as much photons as you can but, but if there was more space infrastructure, perhaps you could match this with, with laser sales at the same time, and maybe you could accelerate, but but I can imagine, the goal is to get out to this, this focus range, but then be able to loiter there. And so if you're going too fast, then you can't, you can't arrive at the destination and stay there, you're going to be going, you know, say you're going 10% The speed of light, then you're going to zip right through that region, and then you're off into interstellar space. So the key is to find that something that will let you get out there, but that also, that be your final orbit, as opposed to being off to another star, Dr. Slava Turyshev: we do not need to stop, Fraser Cain: just keep going observe as long as you can, right? Exactly. Because
Dr. Slava Turyshev: of this, the purpose of this wall gravitational lens, it doesn't have a focal point, it was a semi infinite focal lie. It's a spherical aberration, essentially, if you if you take a spherical lens, you have a spherical aberration, essentially, that object will have a semi infinite focal line. So you don't have to stop, you
just continue along that line. And you can image the same planet with even better resolution why? And with with actually better sensitivity, why because solar radiation, I mean, the solar plasma that the solar corona is a significant noise factor, the further you are from the Sun, the less solar corona will influence the data measurements. So essentially, the further you are, you will be sampling the solar corona, because the signal comes on top of for Corona, it's a major noise factor. And so this is why you need to integrate your pixel integration time is roughly anywhere between 30 seconds, if you're talking about nearby planets to probably about three minutes, five minutes. So for the for this planet, it's a perfect so but no, no, you, you need to multiply by number of pixels, you wanna you want to image the planet. And you need to move from pixel to pixel. Because that planet that we
discussed our Earth, which is let's say situated at 100 light years away, the image of that planet will be projected into a cylinder with a diameter of 1.3 kilometers. Now you need to position your spacecraft and assume that cylinder and move along there are sort of axis of that cylinder. And so within 1.3 kilometers distance, and so that's going from pixel to pixel image that are your image that object and then you take the time series and you process it and recover images, we have shown how it can be done. Fraser Cain: So it just gets better and better over time. So yeah, those first images that say 500 au, and then as long as you stay in that cylinder, targeting whatever planet you're looking at, is there some point where it does fall off? 1000s? Of of a US 10,000 A use it? Or is it as long as you stay in that cylinder? Which I guess is? Or is it a cone that is narrowing as you get farther and farther away from the Sun that you need to remain in that in that it's actually Dr. Slava Turyshev: open and it's not narrow in its opening. So the diameter of that planet will be 1.3 kilometers at the
distance of 650. Au, but it will be now 2.6 kilometres at 1200 AU. The you are opening up and so essentially, but that actually helps you to remove still, the spherical aberration, definitely you are you're not not only you're sampling less of the solar corona in your data, but also your image has now expanded. And so you can actually have a better quality image that the challenge there of course will be resource dependent because we will rely on electric propulsion we have a small spacecraft with a one meter telescope that will be moving in the image plane image plane at any given distance heliocentric distance will be different image plane, so but we can actually process it. But the point is we need to have as we move with the high res we continue to move with a very large heliocentric philosophy. So to remove receeding from the Solar System, we need to move in the political direction. And so
that promotion and the political direction will be enabled by electric propulsion on board. And so those systems that are that are needed for that motion are already available. So the industry already offers first type of center thrusters. So no new technologies are needed for that for that development. So realistically, electric electric propulsion will be used twice.
You mentioned here you mentioned overflow maneuver. We actually in the initial analysis, we didn't use oberth maneuver flying by the Sun we basically just open up sales when we need to reorient sales. And when we come close, the gust of solar radiation pressure will move us. But we can actually implement
Oberth maneuver we can use, let's say maybe electric propulsion block during the solar flyby that will accelerate will give us maybe two or three or 5 million per year velocity with a bit more. So we can we can, we can do that. But then, once we are out there at the focal region, we essentially will be using electric propulsion to move from pixel to pixel in the image plane. And I guess your viewers probably will recognize another another challenge is navigation because at that point, remember, it's a 650, you pretty much it's far away, but we need to move within the cylinder of the diameter of 1.3 kilometers, can that be done? And people will say, No, it's difficult pulsar, you can do it. No, it's even better
solar gravitational lens gives us a very good guidance signal, because I see big because the amplified light from the whole star will be out there. And so you we can use and we don't need to deal with solar corona, we don't need to deal with anything amplified light is there. And so by the study and evolution of the Einstein ring, formed by the amplified light of the whole star, we know exactly how far away we are from the whole star optical axis. And we know it knowing the orbital elements of the exoplanet we know exactly where we will need to be at what place and how long do we need to integrate. So there is a
guidance signal that SGL gives us we use the amplified lights from the whole star to form a local reference frame. Fraser Cain: So you're looking at the shape of the Einstein ring. And as it starts to get a little out of whack, you know, to change your your position slightly to get it back into focus. It's kind of like it's kind of like focusing a telescope. But in this case, you're moving your spacecraft to get back in focus.
Dr. Slava Turyshev: It's it's, it's something like this when you are exactly so the image of the exon, above the whole star, the whole star will be will be a very bright image shows the big cylinder. So when you in the middle of that a cylinder, you will have a fully formed Einstein ring. But if you start
moving away from that cylinder, if you're away from the cylinder, you understand ring initially breaks into parklets, smaller clips, and then there will be two brights plots. And we know exactly how to calibrate the brightness of the spots, depending on the on the spacecraft distance from the optical axis. So you know that the shape the morphology of the of those arc lights, and the brightness of those targets will tell you exactly how many does how many 10s of meters away from the center of the optical axis of the whole star. Now knowing the orbital elements of exoplanet you can pre compute with where this exoplanet will appear. Because ultimately, we
need to be there where the exoplanet is in track track its motion. So essentially, by tracking the motion the whole star with another small spacecraft, we can actually form that local reference frame and know exactly where the hostel where the exoplanet will be and was start moving within the image formed by that exoplanet. Fraser Cain: The limitation obviously, is that you get one target that you one one telescope per target. But if
it's just a meter telescope with a solar sail, like it sounds like a relatively inexpensive mission, would you foresee sending many of these out there? Dr. Slava Turyshev: Fraser 3.7 arrays, before answering your question. I think in exoplanetary community really recognize that the era of surveying missions, probably will be over soon, because you need to increase your you know, there will be a targeted mission missions come in. Because to observe every star in the sky, you can't because there is no enough mission time. So essentially, you need to have targeted observations quickly because you need to observe one target another target. And so there are a handful of targets you have to identify before you start observing before you launch, so initially now with missions that you have, we are discovering planets by observing a particular star multiple times. And so but we cannot absorb infinite number of those
things, right. So there may be, let's say maybe 150 of those targets, maybe maybe 1000s of those targets we observe and then basically, we start to reabsorb them over time, the more data we gain the So, for example, for streaming, using last commit methods of the usual astrometry to detect exoplanets you have to identify maybe 20 point 20 stars only. So it It's not a surveying mission. Essentially, if you want to do a symmetric detection of exoplanets, we are talking about very small, small number of targets. So we cannot observe
anywhere, we cannot observe every star in the sky. So Gaia was the mission that the European Space Agency launched to do astrometry, global assymetric reference frames. And so essentially, Gaia observed billions, billions of stars billion stars. But for searching for exoplanets, we're not going to be absorbing that number of stars. So but those objects are not. It's only indirect confirmation of exoplanets exist around that star, once you will start putting your resources into observing a particular set of targets, he immediately talking about fewer and fewer targets in your mission. So
we're talking about maybe, maybe 20, maybe 25 targets, because now you identify a particular target of interest, and you want to spend more time with the target studying spectra studying composition of certain orbital elements of the target. So it's not, it's not the full price, the radian surveying mission anymore, right. So with the sole gravitational lens, we will not fly this mission to detect exoplanets because we need to have a good target to start with. Because our field of view is extremely narrow, we took in about 3.5 arc seconds. So it's a
very narrow field of view, we cannot change in that field of view on a different set on a different target, you need to move laterally quite significant amount of quite a lot. So we are not planning on moving laterally, we basically need to have a very well determined target. But then, as we, as we see over and over with planetary missions in the Solar System, when we fly to Jupiter, we observe not only one satellite of Jupiter, reabsorb the whole family of satellites around Jupiter, same with with, with Saturn, Galileo and Cassini Spacecraft observed and detected multiple new satellites. In the case of solar gravitational lens, it's a single system mission, where there will be several planets orbiting that particular as a whole star. And we'll be absorbing all of them,
and maybe even the their moons, their satellites. So that's sometimes something unique. And if you've identified a high value target for us, that I think is the best way to deposit resources. And if the mission is affordable, yes, we can contemplate sending several of those missions to to observe images of very interesting targets. And soon I believe with
James Webb, picking up the intensity of operations with James Webb will probably see more targets detected with the test and operations and more missions coming from European Space Agency, within the next five, maybe 10 years, will, the zoo of exoplanets will increase from what now it was about 9000 planets will have probably up to 40,000 planets, and some of the targets will be extremely interesting, because we will be able to observe their spectra, and there will be some interest special interest in spectral lines. But remember, those will be indirect detections for the terrestrial planets, we will not we will not be able to see them. And so at some point we'll have to decide are we going to be building larger and larger telescopes going to 16 meters, 24 meters 30 meters, still absorbing that blob of, you know, part of this space where this exoplanet is orbiting without seeing it directly. Or maybe it's time to really contemplate sentience or gravitational information to stop absorbing that target directly. And so it's a, it's a single system mission, but that will, that will allow us to study the planetary evolution within that particular system will be we will be able to detect our science of the bio signatures on the surface absorbs all of those planets. And that I think this is the way to think about this, because ultimately, the most interesting targets will have to dedicate telescopes to absorb those most interest in targets anyway. And so solar
gravitational lens will come when we have a very good signal saying that this is the this may be the Earth 2.0. And then we need to look at this target very specifically. Fraser Cain: So James Webb will be the finder scope for the solar gravitational lab. salutely. Yeah, absolutely. Unknown: And we usually we appreciate the significant community efforts, because the more targets we discover, the more pressure will be on the community to make the decision what to do next. And realistically that sort of
diffraction limit out the tyranny of diffraction limit will, it's already huge and hard because we build an ever larger telescopes, the largest telescope in our built 39 meters in Chile, right? So the extremely European Extremely Large Telescope, and so there will be 30 meter telescope potentially within the century we can expect seen 50 meter telescopes on the ground, but in space, probably 6.5 meter that is, as per 2020 recommended will be flown in the next maybe 10 years. But then the next will be maybe 12 meter telescope. That's all right. Fraser Cain: What's the other 90,000 we talked about earlier? Dr. Slava Turyshev: When you tried to merge those numbers, the numbers are unmatchable, and realisation interferometers people asked me, okay, we can do interferometers, maybe two you interferometer. We have two telescopes orbiting the Sun,
separated by two astronomical units, wonderful, we'll get probably resolution, but we will never be able to have imaging capability of the field aperture telescope with a large diameter. Fraser Cain: So when we think about us surrounded by all of the stars in the Milky Way, and as you said, these this cylinder of, of magnification is sort of extending out from every star in all directions out to infinity. And I know we do gravitational microlensing all the time to discover planets in the first place. But is there a way to use this technique that you're talking about, to try to catch some of these natural lenses forming just by by stars happening to line up perfectly in ways that we could find useful Dr. Slava Turyshev: of the keys of dynamics, because if everything is static, if all the targets are nailed to the sky, and so then they don't move, then optical access of those lenses will be fixed. In reality, nothing is static in
universe, everything moves, their promotion, their orbital motion, there is a lot of interest and dynamics involved. So those microlensing events, they do happen. Usually, they're not very predictable. And so when they do happen, the last for about a month, when the brightness of a particular store get brighter and brighter for two weeks, and then it started diminishing and never repeats. So in this sense, what we do with for gravitational lens, we engineer that alignment, and we stay on that alignment all the time. And so that's the that's
the difference. Because to do the imaging of any object you need to be aligned to very high precision with the object to be able to still be in within the focal region is geo Fraser Cain: Sure. But I wonder if, if there's a way to take advantage of gravitational microlensing events better, because right now, it's kind of astonishing. Like even amateur astronomers can use their backyard telescopes to observe the brightness of a star and contribute their measurements and confirm the existence of of planets. It's, you can see the
power the raw power of these gravitational lenses as they happen. But as you say, they happen very shortly, you get a month, and then the planet this this one time alignment is over, and maybe you've detected a planet. But is there a way we can take advantage of gravitational microlensing? Through science through observatories through techniques to learn more, as the lensing event is happening? Obviously, you won't be able to stay in line and observe this, this planet forever. But is there something that we can do to take
better advantage of the gravitational microlensing? That's happening all around us all the time? Dr. Slava Turyshev: See, my take on that is there will be limited advantages are to do that the limited advances actually. The reason is that with the Sun compared to the Sun, we know the optical properties of the Sun solar gravitation lens very well, we can remove the properties of the lens from the data and the dealing with true image. Within the case of micro lensing, we don't really know the lens property. We know that
event is happening but what is actually the lens and body or lens in galaxy, how that mass distributed within that lensing galaxy. It's model dependent it's not really well understood, we can confirm that there is some morphology in the image but to attribute that morphology of the image that we temporarily have possession for for four weeks only, we don't really agree we cannot did use a very high resolution information about the about the source source but object so this is where the challenge is because we deal with these two unknowns that are just aligned by gravity. First unknown is the is this object the second unknown is the lens itself because lens may have been a maybe usually lenses astrophysical lenses are not spherical. They may be galaxies were elliptical galaxies. And so when we deal with with elliptical galaxies, the point spread function of those objects as well. really
complicated, and really trying to remove that points per function from the data trying to deconvolve. It's not that simple. Fraser Cain: So even if we had, I mean, obviously, it's not that simple. But even if we had like, I don't like a Vera Rubin
telescope that was scanning the entire sky, both searching for gravitational lensing events is and characterizing the lenses and the objects better and better and better. It doesn't feel like it's a, a useful path to take. Dr. Slava Turyshev: No, because the lens itself appropriateness of the gravitational lens is typically not very well known.
And to remove the artifacts and lenses, artifacts from the data, is it's challenging, challenging, at least, if not impossible, until realistically to assign the the high confidence in the data on the image in particular object without knowing them. optical properties of the lens is with a bit of exaggeration, Fraser Cain: right? I was hoping the Universe had done our engineering for us. But clearly, we can't, we can't get all the advantages from them. Now, but Dr. Slava Turyshev: go ahead, sorry. But then maybe we need to
think about not a Dyson Sphere. But something like you know, Einstein sphere. Well, we have a sphere of spacecraft orbiting the Sun at 550, maybe 650. You on the sphere, you didn't get every object in the galaxy, by using the Sun as the lens. So that's our global observatory. Of course, it's not for now, but with the technologies that may that have been developed, maybe at some point, nuclear fusion, or nuclear, nuclear propulsion.
And the Volvo come online at some point in the future, maybe that's will allow us to, to do that. For now. We are limited with propulsion. Fraser Cain: Yeah. Now, now, this is more than just an idea.
You are one of the phase two recipients at phase two, right? For the night for NASA's nyac Grant. Phase three, phase three, phase three. Okay, great. So that's the you've gone through three iterations now of developing proposals, working on ideas, getting feedback from NASA. Where does the project
stand today? Dr. Slava Turyshev: Thank you for asking this question. Our This is our final year or phase three project will be done by the end of September this year 2022. And at the end of this project, we are aiming to transition from the nyac paradigm on NASA innovative advanced concept Institute where we are very thankful for support. And but realistically now we need to hit the ground running and we need to develop the mission. So we are building the technology, the set of Technology Demonstration Missions that will allow us to demonstrate for sailing as a capable propulsion. Once we have that, we have the spacecraft that is already been, you know, designed well with to survive the mission, the duration and to be able to operate. So realistically another technology
that you need to develop it's not only propulsion, but also power. And so we have a very good concept for distributed power. It's a small nuclear, sort of the elements around around the spacecraft, not a single piece, but a small subset of those elements. And so essentially, this concept, the propulsion and the power, once we address this to I think the mission can go, Fraser Cain: you would need some some version of like an RTG, because you're so far away from the Sun. So it has to be
decaying but but you're saying that you would like break it up. So instead of having like one RTG generator, like say the Voyagers you would have several of them, Dr. Slava Turyshev: there are two different ways of launching the mission. One way is to have a very, when we deal with the soul soul sailing spacecraft, the metric is the performance metric is the area to mass ratio area of the sail to the mass of the spacecraft. So the larger that area to mass ratio, the larger will acceleration, you can actually, you know, the largest velocity you can reach. And so the larger the solar radiation pressure impact on that sail, so but now, you either built very large sail, so we're talking about very significant sales structure, which is now getting into dynamic control deployment mechanism stability of that will build smaller spacecraft. So potentially, we realize that our
mission capable spacecraft will have a weight of roughly about 80 kilograms. And so with 80 kilograms, we can deliver full mission. It's the kilogram, it includes the telescope and meter telescope propulsion block communication will use optical communication for communication purposes. And so that is possible to be launched in one piece but to do that with the technologies I present today, we need to develop sail materials to be able to come closer to the Sun. So another way to do that it's not flying a single spacecraft with a single, large sail, but instead flying maybe two or three smaller spacecraft, and do once you pass by the Sun once you accelerate those smaller spacecraft to a larger velocity than just aggregating flight, and so you already kick your spacecraft to near that velocity. And now they came close to each other, still maneuvering the source and they can sort of compensate for relative velocity, they can come and dock and doc in the spacecrafts, you actually are forming that larger spacecraft.
And that will be moving with very high velocity. So essentially, this is the two technologies to two different paths that we take. Fraser Cain: So let's imagine let's fast forward, you finish the phase three, NASA decides to turn this into an actual proper mission. Some of the other pathfinding technologies have been worked out, we're five years down the road, maybe 10 years at the most. And and the the mission is on the pad. What? How will it work? Like how will sort of the whole mission unfold from this point? Dr. Slava Turyshev: Are you asking about the flight trajectory, I'm Fraser Cain: imagining, like, you know, you've got a you've got a starship, you've got, you know, you've got as you can, because you have to launch towards the Sun, obviously, that takes a tremendous amount of delta V. body could probably
handle it. So you've got a few 1000 kilograms and payload for your spacecraft, if you want, how would you sort of see the whole the whole mission unfolding from that point forward. Dr. Slava Turyshev: So for proper duration in the slow
gravitational focal region, we need to have at least three small spacecraft, three spacecraft to 80 kilogram weight. So So why do we need three so one spacecraft will be our measuring solar corona specifically and subtracting from the data because we need to remove solar corona to have independent measurement of solar corona to remove it. And so it can be done one of the three spacecraft anyway. So basically,
so that is, the number of three is because another spacecraft will be established and local reference frame, remember, we discussed that local reference frame. And so those three spacecraft and interchangeable they can move in, in and out, do the same job. And basically, they all will be able to independently communicate to the ground using one meter telescope and maybe a 10 meter telescope from the optical telescopes on the ground. And so what what else is needed there. So essentially, our once we have those spacecraft as sitting on the launch pad, and so essentially where they will be launched to high Earth orbit, and high Earth orbit, they will open the sails and start moving towards the Sun by sort of reducing kinetic energy. Yeah, so the development capabilities, they will be flying by the Sun in about three or four to five months. And then they will reorient the sails and will
start picking up very large velocity, radiation pressure. And so acceleration from the solar radiation pressure will keep them very strong, so that by the time when they're going by five US clinical units away, we know exactly where they're going because we can trim all the navigational errors and so we can inject them on pretty much ballistic trajectory all the way to the focal region of the solar gravitational lens. If you need to compensate for residual orbital uncertainties, we still have electric propulsion in flight and then we just coast along that focal region to 25 years there plenty of interest in science to be done. So now, now we are focusing on for gravitational lens enabled science right so imaging of exoplanet but there is a lot of interest in secondary science the way we see it, studying the Solar System, maybe flying by several distant objects in the Solar System in Kuyper belt, studying Solar System magnetosphere local, local, medium, and so, there are lots of interest in science as we fly there once we get there. Once we start reaching Riri region of 550 stomata units, this three spacecraft will be able to observe the host star already immediately because now the light is amplified and all three will be able to see her start with no problems there is silk noise ratio in one second more than I think it's more than 10,000. So essentially the light is very it's very bright. And so now they will be able to track the
light from the horse star establishing local reference frame and is the as they move from 550 to roughly 500 feet just 650 you so the local reference per frame will be about well this time blushed, and by the time the Einstein ring will be well separated from the solar disk and the daytime by 651, we will be able to start observing exoplanet because exoplanets will be separated from the solar disk. And the solar arrays for plasma will not be a significant source of noise. And we will be able from 650, the science operations will begin from 650 to 900, we will be still moving roughly 20 to 25. Au per year. And so for the next 10 years, we'll be doing science declarations, we'll be moving this free spacecraft we need the three spacecraft are to compensate also for the unit rotation because the planet rotates. And so to be able to
remove that they will not rotation, we need to absorb it and fire temporal cadence and essentially that will be done while also the three spacecraft will be absorbed and exoplanet. And so they will be coming in the image moving out from the image but they will be doing sort of slow slice and in the different pieces. And ultimately the image will be formed. Depending on the target the image may be formed within three to six months. And but we're talking about the unification
during rotation comes from the fact that or the planet rotates. And in this case, we'll never be we will never see the planet full disk it will be the phases of the planet. And so when we deal with phases of the planet, the images are formed within a few weeks all the way. And so within few weeks, essentially, you will be able a built in Portal set of data to be able to then remove cloud cover, I remove that I only have to do rotations median on the because of the or the dial on rotation on the image. And so we will be moving in that focal region for the next 10 years collecting data LSPs we discussed at the beginning of this conversation, the longer we observe, the better the quality will become. Because we have a lot of data we need to translate transmit the data to the ground, we're talking about kilobits per second, we don't need higher, I mean, kilobits is as much as we want maybe even less listen to a little bit because ultimately our data is basically brightness of Einstein ring on the image sensor, that's all. And so we
move into data moving moving, moving the data down to the ground, and then what you use in the convolutional algorithms to recover the image. Fraser Cain: What do you think is the biggest technical risk right now what is the what is the big thing that you would like to figure out to give you a lot of confidence that that this will work that that it will fly, Dr. Slava Turyshev: multiple missions are going to larger heliocentric distances identified sort of very well known challenges. And those challenges will be power communication, and longevity. So in this case, I guess I will also embrace this the power we need to have very confident source of power, we know how, how, how it can be built. Now
the technology readiness of that approach is about zero three, we need to build more confidence in building this type of distributed power on the spacecraft. That's 1.0 i Fraser Cain: Sorry. So just because the with the RTG it's it starts to degrade the moment you launch it, and you're losing say 25 years of power before you actually get into science operations, right. Dr. Slava Turyshev: Yeah, and the challenge there of course, is we need to have less power hungry instruments. So that will not derive drive the power available quite significantly, but still beginning of life will be a lot of power, then we need to dissipate that power in flight because we will be accumulated and batteries on board and then beginning of science operations, that will be the key metric, how much power do we have at the beginning of science operations after 25 years of flight, and then we still we are continuing to be be we continuously moving in the image plane full of the optical axis the image of that star of that of the exoplanet. So, we
stressed an electrical thrusters will be most the the most power consumption will be due to due to electrical thrusters, because we move a lot. And so by moving very using power and then the another key metric is how much operable you have by 900 a year. So we are designing the power system such that will allow us to have reasonably confident comfortable margin by the time we reach 900 a year. So that's something is important. And we would like NASA to pursue this technology that we developed with distributed power resources, which is integrated with radiation hardened battery. And so that's one thing. The
second is of course, I mean, the challenges that we identified and so that is think about this, we never built in mission that will have to survive 40 years of life. Fraser Cain: I mean, we have missions that have survived 40 accidentally at to gently avoid your wagers, etc. Yeah, yeah. Dr. Slava Turyshev: a wonderful example. Words are a wonderful Playing here's example. But they were built to last maybe 3.5 years. And they will designed really simple, right and the way built to operate extensively very well. But there were as simple as brick, I'm sorry, I'm sorry to say that that as simple as possible, there are nothing can be broken. So the mission
designed for this definition needs to change the design paradigm, essentially, because we have to know engineer for longevity. And so as you know, electronic components, they're not really loans on leaf. So because the more electronic components we have, the less the more likely they will go go go berserk on you. So realistically, longevity, and then contamination and flight. So this is a topic that
uniquely, uniquely came into the study of the soul gravitational lens, you need to address not only classical challenges, which is communication and power, but also longevity and, you know, mission organization. So essentially, in the history of humanity, only few projects, that lasted more than a few years, few few decades were unknown, for example, great wall of China, built over centuries of you know, people were building that egyptian pyramids, right. So it's basically different organization, the differences, the different societal organization, and different objectives. But here, it's a mission that needs to be developed, launched, flown and separated by the group of people who will be dedicated to the total work, that is something we had never done never had never done before. But that is interesting. Fraser Cain: It has, I mean, that has value across everything that NASA does. I mean, imagine if the Voyagers were built with
an intentional lifetime of 50 years, right from day one and not three years. Dr. Slava Turyshev: Absolutely. And so everything that we discuss with solar gravitational lenses pushes pretty much every element of mission design because we need to have reliable components reliable operations, autonomy, because we cannot drive the spacecraft by sending back and forth commands from from the ground to spacecraft, it must be we must be able to cut umbilical cord, because any mission that we operate today at NASA, that umbilical cord from DSN, you communicate. When the rover on Mars, pretty much daily can we communicate with our own daily telling here, they're telling the robot what to do with a mission like that, we have to cut the umbilical you have to trust the system that will be it will have to operate autonomously and have to compensate for any anomaly that is occurring happening on board and they will be anomalous, but it should be able to sustain a normal interview right. So this is something conceptual is very different, we need to trust our robots more than we used to. And so that changes significantly,
the way we operate our assets in space. Fraser Cain: And similar challenges being faced by the team that's working on the interstellar probe, same kind of distance regime not quite as far but but that same need to have a very long cruise phase before you before you and you will need to have your spacecraft still still operating. And I think really the unexplored parts of the Solar System. They are the DIS the more distant places, Uranus, Neptune, Pluto, the Kuiper Belt, that all of these needs to be this shift towards longer lived spacecraft that can still be in middle age and doing its, its work. And if we ever do want to send a spacecraft to
another star system, then we're looking, even if we're gonna go at a significant portion of the speed of light, we're still looking at decades if not hundreds of years of continuous operation. And that takes a completely different paradigm of thinking. Dr. Slava Turyshev: Exactly. And to think about solar gravitational lens is the first small for us to close that creek between the planet Earth to nearby stellar systems. So that's a way of wandering outside the Solar System to a very specifically identified region, this region is very well studied by now. So the sole gravitational focal region. And
so by doing that, we essentially will have to develop technologies that will enable us to move further and that is the most powerful realization that as we build solar gravitational lens and it will push every mission design every technology beyond the current limits. And so we do not see a showstopper, BBC challenging development, but exciting technology development that is based on the technology we have today. And so I didn't mention also Thoris for sailing, because we saw sailing with the technologies that we Technology Demonstration Missions that we are developing now. We should be able in about three years with our industry partners to start to start flying those T DMS that will be flying with faster and ever faster velocities and once we do that Imagine what we can do with this fast transits through the Solar System. Because it can be those
technologies can be those spacecraft can be used as drones to study the Solar System. With velocities reaching velocities of seven to 10 astronomical units per year, at a cost of roughly 15 to 20 million per spacecraft 20 million, it changes the paradigm of solar system exploration. Now we're talking about long lived spacecraft, we can study distant satellites of planets, or objects in the Solar System, with the cadence much faster than once per 20 years, we can do this every year, several of them and especially the course we're talking about, Fraser Cain: you could imagine a starship loaded with 100 of these, and then it just, it just throws them towards the Sun, each one goes off to a different Kuiper Belt object and gives us a proper survey of the Kuiper Belt or the the Trojan regions or, or anything out there that's of interest to us, Dr. Slava Turyshev: exactly in here, within the inner solar system would be maybe actually within the Solar System, we don't have to rely on optical or communication capability, we can shape the surface of the sail in the parabola and use microwave radiation basically, to communicate with the Earth using deep space network. So because we have a very large sail on the spacecraft to start with just repurposing the sail after we went through solar perihelion, you can use that sale, maybe be dropping part of it and leaving, let's say, tip 10 meter diameter on that on the on the sale craft, you can use 10 meter antenna in space, communicating with microwaves back to the ground. And so now you have very capable spacecraft is actually very light, inexpensive, going in a very different places. And
we can, you know, use go go and by, you know, Enceladus, Europa. And they've just taken the first data in situ measurements, not crushing them in the in the in this divide is, of course, but actually having some in situ bio signature detection. And we can go that much faster rather than waiting for those missions for flagship missions every 30 years. That's that's the change.
Fraser Cain: Well, it's absolutely fascinating. And I really hope this happens in in both of our lifetimes. If people want to keep track of what you're working on, what's the best place to do that? Dr. Slava Turyshev: Well, at this moment, may be contacting us directly. And so we'll be talking, there will be a
conference breakthrough discuss this year in June. So we'll be talking about this. This set of missions that we I discussed a few minutes ago, the first transit through the solar systems, through the Solar System, we'll be talking about those will be a separate session dev
2022-04-23 17:29