The Transistor That Won the World
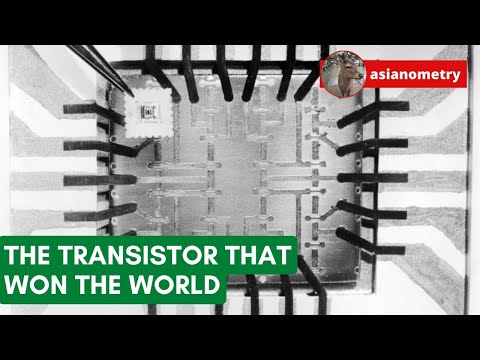
In the early years of microelectronics, there were many types of transistors. But one rose up to rule all the others. Based on an idea that physicists have chased for decades. And today, we have made more of it than anything else we have ever made in human history. In this video we talk about the MOSFET - the transistor that won the world.
## Beginnings Before we begin, I want to recommend a fantastic history book on the MOS transistor - "To the Digital Age", by Ross Bassett. It wasn't the only source I consulted for this video, but it was by far the best. Okay let's roll. The key idea behind the MOS transistor is the "field effect".
Simply put, the Field Effect describes a solid's ability to change how well it conducts a current when in the presence of an external electric field. Physicists have long known about the field effect basically since the 1800s. However, no one had yet been able to understand and build devices to take advantage of it. Notably, in 1928 the Polish-American physicist Julius Lilienfeld filed several patents for solid-state devices, including a potential field effect device.
The patents describe devices that are kind of similar to modern ones. But Lilienfield lacked modern theoretical knowledge and techniques. So the devices could not have worked. His patents did not attract much attention at the time. A few years later in 1935, the German physicist Oskar Heil files another set of patents for a more advanced device. However, same as with Lilienfeld’s patents, there is no evidence that a real device was ever made.
I wonder then if such a thing can even be patented. But they also gave Amazon a patent for buying stuff with one-click so who knows. ## The Missing Field Effect While at Bell Labs, William Shockley was in search of the field effect. He, like the rest of Bell Labs, sought a solid-state device that could do the work of the vacuum tube. Back then, the tube was the dominant device for switching and
amplifying signals. But its unreliability incentivized people to find an alternative. In mid-1945, Shockley and his partner Gerard Pearson crafted an experiment to try and create this device. Here is how it worked. You had a layer of semiconducting material, laying on top of an insulating support. And then set very close to that semiconductor layer - separated by less than a millimeter - we had a metal capacitor plate. In the experiment, Shockley applied a voltage to the metal plate. If all goes well,
then a strong current should flow over through to the semiconductor material. However, when Shockley ran the experiment he found the actual effect to be far weaker than expected. This puzzling discrepancy led theorist John Bardeen to suggest in March 1946 that there were traps on the semiconductor's surfaces keeping the electric field from affecting the rest of the semiconductor. This led Shockley, Bardeen, and experimentalist William Brattain down the path to the point contact transistor - the first transistor. ## The First Transistor I covered this story in a prior video but we should discuss it even if passing.
Bell Labs' 1948 announcement of the Point Contact transistor set off a flurry of research. But that particular transistor was not commercially viable. It consisted of two metal contacts barely touching the surface of chemically treated germanium.
For this reason, they were difficult to build and easily broke. Worse yet, people did not very well understand the physics behind how it worked - which made it hard to build upon. William Shockley pivoted away from his Field Effect ideas and towards what he named as the “minority carrier injection” concept. And then based on that concept, he creates the bipolar junction transistor. ## Bipolar's Rise The bipolar junction transistor is basically a semiconductor sandwich. The first such transistors were made from a single crystal of germanium with three sections - an emitter, base, and collector.
The emitter and collector would be made from the same P-type or N-type doped germanium, but the opposite of that used for the very thin base. So there are two barriers or PN junctions - between the base and the emitter and the collector. The "bipolar" in the name refers to the fact that when the device is in operation, both electrons and electron holes travel through the base. It took some time and precision to actually make this device. But finally in 1950, Bell Labs' Gordon Sparks and Morgan Teal leveraged their crystal-growing skills to produce these bipolar junction transistors.
The bipolar junction transistor very quickly replaced the point contact transistor as the era's dominant transistor technology. It was far sturdier and we better understood its electrical behavior. But it was still a tricky thing to make - particularly the crystal-growing techniques to produce the very thin base layer in between the emitter and the collector.
Only one such base could be made for each crystal, which then had to be cut out and manually located for processing. It was both kind of wasteful and also very manual. Nevertheless, the bipolar junction quickly became the dominant transistor of the 1960s. They were the transistors used for the first transistorized electronics goods like hearing aids, computers, and radios. ## Alloy Junction and the JFET In 1952, General Electric announced a way to simplify the bipolar junction transistor technique. This ingenious technique had you alloying two very small pellets of indium - about a millimeter large - on opposite sides of a thin slice of germanium. You can align them with dots.
This worked well, and for a brief time alloy junction transistors were the dominant transistor technology of the age. It also inspired Shockley to go back to the field effect idea. In 1951, he came up with an idea. What if we were to use a similar set up to "pinch" off the flow of a current passing through a semiconductor? You create a semiconductor bar with a source on one side and a drain on the other. In between you have the channel and a pair of gates on both sides of that channel. When you apply a certain voltage to the gates, it grows what is called a depletion region in the semiconductor. A depletion region
is sort of like a void with no electrons nor electron holes and so acts like an insulator. A higher voltage can pinch off the flow of electrons or electron holes - depending on the material - passing through the semiconductor. Just like how you can pinch off a hose with your fingers. Shockley passed this idea to two team members to work on - George Dacey and Ian Ross. That last name ring a bell? Ian Ross was a student of Charles Oatley, the scanning electron microscope pioneer at Cambridge! I covered him in a former video. Ross later became the president of Bell Labs. The "Semiconductor Shared Universe" grows. Dacey and Ross worked together and in 1952 eventually created the Junction Field Effect Transistor or JFET - the first practical field effect transistor. The thing worked exactly how the theory said it would.
But Ross recalls that it did not work better than the bipolar junction transistor nor was it any easier to make so they set it aside. The JFET as it was so called would later make a resurgence with the rise of silicon carbide technologies. ## Passivation There was a reason why the Bipolar Junction Transistor worked so well for people like General Electric, Bell Labs and Texas Instruments at first. John Bardeen had been right. The existence of traps on the surface of the Semiconductor
did indeed cause the field effect to not work as well as it should have. The bipolar junction and the junction field transistors worked so well because electrons or electron holes are traveling through the entirety of the material. This way, we can somewhat bypass these troublesome surface effects. But the surface effects do still exist. We can't just ignore them. They are worth
studying. And in the late 1950s, a peculiar pair at Bell Labs figured out something important. Mohamed "John" Atalla was born in Egypt, and graduated from Cairo University with a degree in electrical engineering. He then received a PhD from Perdue University and joined Bell Labs after that. While studying the surface effects of silicon, John discovered something. The reason why the surfaces of semiconductors like germanium or silicon can "trap" electrons or electron holes is because they have what are called "dangling bonds". These dangling bonds grab electrons like how carnivorous sundew plants grab flies and eat them - interfering with the semiconductor's electrical properties in the presence of an electric field.
But. If we are to grow a layer of silicon dioxide on top of the silicon using heat, oxygen and water, we can "passivate" these dangling bonds and prevent them from messing with our electric vibes. So passivating silicon with silicon dioxide stabilizes the surface for electrons or electron holes to travel along it like asphalt for a highway. Atalla's work on silicon surface passivation eventually caught the eye of a guy named Jean Hoerni. Jean Hoerni of the Traitorous Eight and Fairchild? The Semiconductor Shared Universe just keeps growing! In December 1957, while thinking about these ideas in the shower, Hoerni parlayed this idea into a fabrication method that used silicon dioxide to protect PN junctions from the elements. He wrote his ideas down and set it aside.
## Atalla and Kahang Atalla meanwhile revitalized some of the designs done in previous years by Shockley and others, and proposed to use pure silicon dioxide as the gate oxide for another crack at the field effect transistor. He recruits someone to implement this idea. Dawon Kahng was born in Seoul, South Korea and traveled to the United States to do his PhD at Ohio State. At this time he had just joined Bell Labs - a new graduate.
Kahng and Atalla leveraged the work of their Bell Labs colleague Joseph R. Ligenza, who in 1959 discovered and patented a method of growing high quality silicon dioxide layers on top of silicon with high pressure hot steam. A precursor of thermal oxidation furnaces. They presented the results of their work at a conference in 1960. They noted its potential usefulness for integrated circuits - then only invented two years earlier.
The thing did not have a name at that time. Their paper simply referred to the notion of Silicon and Silicon Dioxide Surfaces - “Silicon-Silicon Dioxide Surface Device". Which points to the key item of the discovery. ## How It Works So why did this device work and all the previous ones did not? Let us go through it. We have the source and the drain. In between them is the gate stack - which
is made from an aluminium gate sitting on top of the thermally grown silicon dioxide gate oxide. These all sit on a substrate made from either N-type or P-type silicon. N-type, meaning that the silicon is doped to have more electrons. In the industry, we call these NMOS. P-type meaning that it is doped to have more electron holes. We call these PMOS. Together, PMOS and NMOS make Complementary MOS - CMOS. CMOS is Asia's breakthrough low-power semiconductor technology and the dominant configuration of all today's big digital logic devices. Anyway back to our simple example. When a voltage is applied to the gate,
it creates an electric field. This field repels same-charged particles out of the doped silicon while also attracting opposite-charged ones. So if the silicon substrate was P-type, then it has an excess of electron holes. We apply a negative voltage to the gate, which repels those holes out of the immediate area while also attracting electrons into them.
When we reach the threshold voltage, there is enough opposite-charged particles brought up to the surface to conduct a current from the source to the drain. This is your channel. The fact we basically inverted the mix of electrons or electron holes in the surface layer of the semiconductor give us the name - “inversion surface channel”, a critical component of the MOSFET. So why did it not work before? The key point was the oxide layer, which passivated the dangling bonds in the silicon surface and made it possible for the current to travel - essentially paving the highway with asphalt.
Furthermore, the substrate silicon has to be very clean, and the silicon gate oxide must be very pure. Looking back at it now, there was no way it could have been done earlier. It barely worked even then. ## Cool Reception You might imagine that this is the part where the world immediately adopts the new invention and everything changes. Not the case at all. The MOSFET wasn't even the main attraction of that 1960 conference.
Far more people were enthralled in an announcement by Bell Labs about the creation of junction transistors using epitaxy, a form of vapor growth. Though I have to admit this was a real manufacturing breakthrough that greatly improved existing junction transistor yield and performance. The rest of the industry rapidly adopted this technique. General disinterest in this early MOS device was in part due to the fact that there were already multiple field effect devices floating around at the time. JT Wallmark of RCA filed a patent for a field effect transistor in 1957, though they didn't do anything with it. Another company called Crystalonics was marketing JFETs. The French had
their own version of it, the Technitron. So on. More importantly, this first device did not appear all that great nor reliable. Kahng's technical memo at the time noted the device's inconsistency from one fabrication attempt to another. This was at a time when the semiconductor industry was really focused on bipolar transistors. Bipolar technology was more mature, and critically, more reliable. If the whole point of the venture was to replace unreliable vacuum tubes, then it sort of invalidates everything if the replacements are just as unreliable. And that was the case with this early device.
And the one big thing that Kahng suggested their device can do - mass manufacture on integrated circuits - was not yet appreciated by the industry. Nobody believed that a large Integrated Circuit can be relied upon without bad yields crippling the device. ## Aftermath So at the time, the response to the 1960 announcement was like "Cool, another proof of the Field Effect" - and then everyone went back to their bipolar transistors.
In part due to this cool reception of his breakthroughs, John Atalla grew discontent at Bell Labs and left to join Hewlett-Packard. There, he helped found their research labs and directed their solid state work. He retired but came back to work on ATM technology, inventing the Atalla Box - a hardware security module that is still used today as the de facto standard. So yes, the co-inventor of the MOSFET is also responsible for your ATM PIN code. As for Kahng, he would stay at Bell Labs for over 28 years. Along with the late Simon Sze, he did pioneering work in flash memory and eventually became a Bell Labs Fellow.
After retiring in 1988, he joined the NEC Research Institute as its founding president but passed a few years later after suffering an aortic aneurysm. ## RCA Atalla and Kahng's presentation in 1960 did catch a few people's attention. One of the companies was RCA, who instantly recognized how the duo's transistor structure had value as compared against Bipolar Junction transistors. First, the MOS transistor structure scaled down well, physically. Bipolar Junction Transistors on the other hand suffered worsening delays as its dimensions shrank. Second, the structure inherently protected its most important part - the gate oxide.
Right after you make the gate oxide, it gets covered up and protected by the metal gate. And third, fabricating MOS transistors required fewer steps than that for Bipolar Junction Transistors. And being an essentially flat transistor, you can potentially stuff more of them onto a single piece of silicon. With RCA in the midst of a major foray into the computing industry, they assigned a team to an MOS effort.
Two years later, Steve Hofstein and Fred Heineman presented the first ICs with these types of transistors. They called them the "INSULATED GATE FIELD EFFECT TRANSISTOR" or "IGFET". ## Fairchild At around the same time, the iconic Fairchild Semiconductor started getting into MOSFETs too. Remember Jean Hoerni? Back in 1957 he wrote down his idea for using silicon oxide to protect the critical PN Junctions of a transistor, cutting holes into it as necessary to add the transistor’s other features.
Two years later he went back to this idea, creating the "planar process" in 1959. At the time, Fairchild produced a different type of transistor called the "mesa", called as such because it looked like one. After applying the planar process, he confusingly called this modified mesa the "planar transistor", which is not the same as the "planar MOSFET". A member of Fairchild's R&D team, Frank Wanlass - who first learned about MOSFETs from RCA's work while he was doing his doctorate - fabricated MOSFETs at Fairchild using the planar process. It worked wonderfully and Fairchild decided to sell them. Side note. During his brief time at Fairchild - just a year and a
half - Wanlass also came up with the aforementioned CMOS concept. Legend. Fairchild's marketing department came up with the MOSFET name in October 1962, though the MOS phrase had been around since 1959 when a guy at Bell Labs named John Moll coined it. When RCA caught wind that Fairchild was about to release a MOSFET, they rushed out theirs too in February 1963. Though neither would hit the market in volume until 1964. RCA marketed their transistors with the IGFET name, leading to a period of time when the industry could not decide between IGFET or MOSFET. MOSFET thankfully won when
everyone realized that nobody knew how to pronounce "IGFET". ## Improvements None of these early MOSFETs in the 1963-1966 period worked all that well. They suffered serious reliability problems - the gate oxide kept breaking down - and the transistors' failed to perform as well as their bipolar competitors. Then in 1966 through 1968, a series of discoveries found that sodium ions were infiltrating the gate oxide after its fabrication, causing it to break down after a few years.
So Fairchild added a silicon nitride overcoat over the whole chip - instantly making the MOSFET as reliable as anything you can possibly get. But there was still one last problem - performance. Many of the early MOS transistor-based integrated circuits still failed to outperform bipolar transistors, speed-wise. They also suffered from high threshold voltages. ## Making MOSFETs The issue was in the MOSFET's metal gates. But to explain that, I first need to explain how we made these things in the late 1960s. Let us imagine again the surface of a silicon wafer.
Nice and shiny like a metal pog slammer. We then grow a relatively thick layer of oxide - about a micrometer thick - on top of the surface of this silicon wafer. This is called "field oxidation" and was done using a process called thermal oxidation. Which basically meant shoving the wafers into an expensive oven with some water and oxygen.
After that, we use photolithography to define where the source, drain, and channel will be. You know how photolithography works? Good, let us avoid that rabbit hole. Once we know where the source and drain will be, we must dope those regions. Since we are in the caveman days before the British commercialized ion implantation technology, we need to use diffusion. With diffusion, we put the wafers into another high temperature furnace. Then we take the dopant - usually in a liquid form - and heat it up along with some oxygen. The dopant will react with and diffuse into the silicon surface.
It amuses me how much semiconductor technology in these days depended on just cooking these wafers in an oven along with some random chemicals and praying that it comes out okay. Literal witchcraft. After the source and drain are defined and diffused, we use etch processes to scrape away a hole in the field oxide layer where the gate should be. Then we can re-grow a very thin, very pure layer of silicon dioxide on top the silicon substrate using thermal oxidation again. This is our gate oxide. And then on top of that, we finally deposit the metal gate via evaporation. By now the transistor is essentially done. Note how in this sequence, the gate is produced last.
## Misalignment So what was wrong with the gate? It had to do with the decision to use aluminium. Aluminium the metal melts at 660 degrees Celsius. But diffusing silicon requires far higher temperatures, 1,000 degrees Celsius.
What this means is that we have to do the source and the drain BEFORE we make the aluminium gate. This requires us to align the aluminium gate right on top of where the micrometer-sized channel will be - consistently. This was hard. In response to this, MOS manufacturers would make the gates bigger than necessary in order to assure alignment with the channel. This led to even more problems because it sometimes caused the gate to overlap the source and drain.
## The Polysilicon Gate As this dawned on the industry, a brilliant new innovation emerged - the polysilicon gate. The "poly" in the polysilicon means that the silicon is very pure, but its atoms are not arranged into a single crystal format. But other than that, it's just silicon. Replacing the aluminium gate with a gate of heavily doped polysilicon let the semiconductor makers make the gate stack first before the source and the drain. Once the gate is built, it can be used to position the source and the drain. And since the gate is made from polysilicon and not aluminium, it can survive the high temperatures of diffusion.
Imagine it as like - instead of trying to fire an arrow at a bullseye target, we fire the arrow first and then draw the target around wherever the arrow hits. The gate being self-aligned to those doped regions gives the technology its name: Self-aligned gate. It was a key breakthrough that both cut the MOSFET's high threshold voltages and increased its speed 3-5 times. It did somewhat invalidate the M in the MOSFET name. Silicon
is not a metal but a semi-metal. But whatever. ## Conclusion Four established firms came up with the self-aligned gate technology at the same time - General Electric, Hughes Aircraft, Bell Labs, and Fairchild Semiconductor. But just like how Google didn't do anything big with the Transformer architecture until OpenAI came along, it took a new startup to fully take advantage of this breakthrough. That startup was Intel, whose cofounder Gordon Moore had been Fairchild's R&D head. Moore saw
the technology in action and then hired many of the technicians who developed it. Self-aligned gates revolutionized MOSFET and in turn, integrated circuit technology. It allowed Intel to ship market-leading memory chips like 1969's 1101 SRAM, breaking into the market. And it set the paradigm for decades of semiconductor scaling. The planar MOSFET would not change until 2011.
2024-06-23 14:53