Technology Day 2022: New Research on Aging and Longevity – Emery Brown
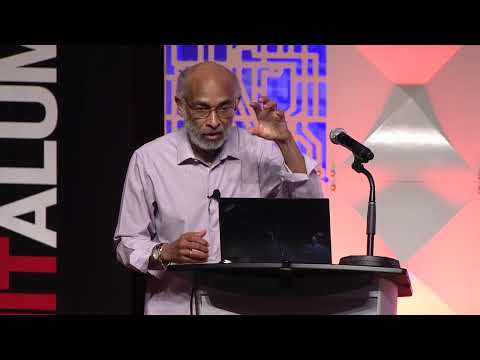
Whitney Espich: Hi, I'm Whitney Espich, the CEO of the MIT Alumni Association and I hope you enjoy this digital production created for alumni and friends like you. So I'm Emery Brown. I had the good fortune of being in the Picower Institute in BCS with Li-Huei Tsai. And I'm an anesthesiologist, in addition to being a neuroscientist and a statistician.
And so what I want to talk about today is anesthesia, all right? So how many people here have had anesthesia? See? I knew it was a good topic, all right? [LAUGHTER] All right? All right? So what is general anesthesia? So it's this drug induced reversible state where you have don't feel pain, you're unconscious, you don't form any memories, and it's nice if you're not moving around while the surgeons are operating. [LAUGHTER] And you have stability and control of the physiologic systems and the key thing is reversible, because if you take those criteria there by themselves those first four, they're synonymous with death, OK? [LAUGHTER] So that's not cool, all right? Now it's often said it's not clear how anesthesia works, and nothing could be further from the truth. And that's what I want to show you here right off the bat. But let me just give you some factoids about general anesthesia in the elderly, all right? So by the time we get to be in our 70s, and I can tell you that I'm approaching-- I'm closer to it than I'm not, let me just put it that way. [LAUGHTER] I mean I got my AARP card more than a decade ago, and Social Security still sends me regular information.
So the likelihood we're going to have surgery is going to be quite high. And 40% to 60% elderly patients, and elderly doesn't have to be-- it can be over 50, let's say-- is going to have some sort of brain dysfunction after general anesthesia. And that could be anything from just having a little delirium when you wake up from anesthesia up to word finding, which could last for several months, all right? And poor pain control, nausea, and vomiting, still the common problems, and it's because anesthesia is practiced empirically. So back in the fall we celebrated 175th anniversary of ether anesthesia at Mass General Hospital. And the most commonly used anesthetic still is sevoflurane, which is an ether. So in many respects we still haven't made a lot of progress.
And what's the missing link? We haven't used neuroscience, and that's what I'm going to talk about today, using neuroscience to actually improve-- And it's really obvious, but it's not so obvious that it's been taken advantage of up to this point. So I'm going to go over 4 ways of making this better. So one is going to be intelligent monitoring. So to give you some sense of what's going on here.
So Li-Huei talked to you about the gamma rhythms, right? And so this is like a brain showing gamma rhythms here all right. And? I've marked two parts in the brain, which are very important for communication, the frontal cortex and the thalamus are two very important centers, the cortex for cognition, the thalamus for controlling all sorts of information, sensory, motor, visual, auditory. And so this is of the thalamus and cortex normally communicating like those gamma rhythms that Li-Huei was talking about, all right, very broadband communication.
Now what do anesthetics do? What they do is they hijack these rhythms. They take them over. So it makes sense from what leeway told us that if the oscillations are there and we need them for cognition, if you hijack those oscillations and you change something like this into something like this it's going to be very difficult to transmit information, all right? And this is what the drugs are doing. So the anesthetics work by causing oscillations which disrupt how the various parts of the brain communicate, and it does it in a very, very specific way. So we could look at the EEG of the different anesthetics like this.
So this is propofol, sevoflurane, ketamine, dexmedetomidine. Maybe you can see that those are different. They have different dynamical patterns. But one of the things that we've been looking at is not looking at them in terms of just the raw data. It's no surprise to this crowd that if you look at them in terms of the spectra you can see the differences in the oscillations quite readily. And so different drugs, which are in different categories have different oscillatory patterns.
Just like you can make a spectrum of my voice here, the drugs have spectral signatures. And these spectral signatures, they're not just phenomenology, they actually relate to the mechanism of how the drugs work. So we could do a very deep dive into how these oscillations come about. And so again, just again, referring back to what Li-Huei was telling us, if we need to have gamma oscillations like this in order for our brains to communicate properly and all of a sudden we bring in drugs which make your brain do this, and then, worse than that, we just let the drugs wear off, it's not surprised that your brain wouldn't work after anesthesia. It's not deep. It's actually fairly apparent.
So one of the things that we should do is we should monitor the brain more carefully. And one of the things I just want to make clear right here right off the bat is, it's no surprise that the oscillation has changed very, very dramatically as we age. So up here in the separate corner here, this is a 30-year-old person who's on propofol. So propofol has a very characteristic signature, a 10 Hertz oscillation, 10 cycles per second, and one at about 0.1 to about four cycles
per second, a slow oscillation. And you can see the two bands there. So this is someone who's in his 30s. So here's someone who's 57.
This gentleman down here could be the guy's father, let's say. You can still see the oscillations. They're little fainter, but they're quite strong, right? And look over here.
Here's a woman who's 81 years of age at the end here. And so this is a lady I took care of who had a tumor on her chest the size of an American football. It took the thoracic surgeons the better part of six hours to remove it, but I was able to give her a fraction of what would be the standard dose of anesthesia, because I'm monitoring her EEG.
And I'm using it to dose the drugs. I'm going to show you that in more detail in just a second. And no surprise, look at this person here in the middle. These two guys are the same age.
His EEG looks like hers. His EEG looks like his. We age different physically. It makes sense that our brains would age differently as well. What's interesting about it is that you could see it under anesthesia.
Now why is this? So forget about anesthesia for the purpose of anesthetizing someone so they can have surgery. Think of this as a neuroscience experiment. We put in a stimulus and the stimulus makes the system oscillate, so if the system is nice and healthy it oscillates like this. As it ages, it has much more difficulty oscillating.
And some of this, we don't have to invoke very gross changes, let's say, it's Alzheimer's plaque formation or these sorts of things. This is just normal aging, because, remember, this 81-year-old lady here has her neurons which have been around for 81 years. And what happens to them over time? The myelin sheath breaks down. The dendrites don't extend and attract as much as they used to. The cell volume declines.
With time, the mitochondria don't work as well. So all the things that are necessary to efficiently transmit the electrical signals across the-- basically, the wiring is 81 years old, and it's breaking down, essentially. So as a consequence, you don't need much anesthesia to anesthetize someone who's an older age, just a very basic idea. And you can look at the kids there. So there's a three-year-old.
There's a 14-year-old. It actually turns out you can see they have the same patterns across age, but what happens is, is that it's the same pattern, but the spectral bands are actually broader. And in fact, the broadest band is in kids six to eight years of age. That's just an empirical observation. Why that's the case, we don't really understand. But the key thing is, we can use this to better dose the drugs, and also-- So you can see the strategy you use for someone, a young kid, is different what you do from an older patient.
And so we've done this now. We've built machine learning algorithms. No surprise that the computers, we can train them to watch the brain much more precisely than we can ourselves. And these aren't embellished pictures that I'm showing you. If we were to go to the operating room now and look at the spectrograms they look just as obvious.
This is information which is not being used. So we've put together teaching programs to train anesthesiologists how to do this, and, at present, may be somewhere around 25% of anesthesiologists use the EEG in some form to actually track the brain stage of their patients under anesthesia. Most of it's still done quite empirically. So this is a place with just education we can help improve care dramatically.
Multimodal anesthesia, again, neuroscience-based. What do I mean by this? So I'm going to say something really obvious, you need anesthesia, because surgery hurts, OK? All right. So as you know we've had an opioid epidemic. Why have we had the epidemic? It's because what we've done is we've used opioids exclusively, but there are other drugs which can be used to control pain. So this is an idea called multimodal general anesthesia, which I've worked out with a colleague of mine from Mexico, Marusa Naranjo. And the idea is very straightforward.
So use multiple drugs to control the pain pathways, not just the opioids, all right? And we can pick from a broad selection. Then what's going to happen is, as those drugs control pain, they also decrease arousal. What that means is the amount of propofol or sevuflurane that I need to give you so you can stay unconscious becomes less, all right? So but to do this have to monitor level of arousal.
So we've got the EEG. We have to what's happening with the pain systems. We use heart rate and blood pressure to do that. And we should continue to do this in the post-operative period. So here's an example.
This is a woman I took care of a couple of years ago. She's 89-plus plus. So the HIPAA won't let you say how old someone is if they're over 89. So she was-- [LAUGHTER] So she was over 89.
Let me just put it that way, all right? [LAUGHTER] And she has diabetes. She has high blood pressure. She's going to have part of a colon taken out, because she has diverticulitis. And there's, you can see, she's not that tall. She's about five feet.
She weighs a little under 60 kilograms. Now I used three drugs to control her pain, dexmedetomidine, remifentanil, that's a synthetic opioid. And I also use lidocaine, the same sort of lidocaine that you think about when you're going to the dentist office.
You can also use it intravenously. So I'm hitting three different pain centers, or three different pain pathways. And the punch line here is in the next line. I was able to give her a 30 micrograms per kilogram per minute of propofol to keep her unconscious. The recommended dose is somewhere around 80 to 100, all right? And it's milligrams per kilogram per minute, and it's because I can actually see what her EEG is doing, and I'm dosing it using this ideas.
And just to show you, this is her vital signs up here at the top. They're quite stable. This is her EEG.
She has just sort of these very large slow oscillations, which is very typical. Like I showed you with the 81-year-old lady. You don't see all the higher frequency oscillations. So it's very straightforward to do. So how does this work? So just a little schema that I put together here just to illustrate this.
So what you do first is you pick from this category of drugs here to control the pain. Use more than one. Let's say, three, like I showed you before. Then that has a very large secondary effect over here. What it does is it decreases arousal, and the practical implication of this is that you don't need as much propofol, or sevuflurane, or whatever to make someone unconscious. So we're actually starting a clinical trial of this at the Beth Israel Hospital.
We've done some patients with this sort of informally. And the idea is we're going to do it for cardiac surgery to really illustrate the idea. But again, we're just using the neuroscience to actually help us think through how to take care of the aging patient. Closed-loop control, all right. In the operating room and also in the intensive care unit, a third idea. So once you've trained the computer, it can watch the brain better than we can.
And you can imagine, as an anesthesiologist, you're sitting there. There are many things that you have to do during the case. And you're going to look away from the EEG data from time to time to see what the brain is doing, but all this should be so we can have the computer watch it to help us, basically. So this is work that I've been doing with my colleague, Earl Miller, who's also in the Picower Institute here at MIT. And it's a control system, all right? So we have a target here, a level of unconsciousness.
We have a way of defining that using the EEG or the local field potentials in the case of the monkeys. And then so what we do is we set that at some level we'd like to have. We have a control system here, a very standard control design. There's nothing sort of out of the ordinary here.
So here is the target that we'd like to have, and over here is what the level actually is. So the controller controls an infusion of propofol, which is going into the monkey, and then every 20 seconds it makes an update of this, the dosing scheme. And so this is basically the closed loop, all right? So how does this work? So I just want to show you this little video. So we've set the marker, the target marker at one, all right? So one is an adequate level of anesthesia. The monkey is adequately anesthetized. And what we're going to do is we're going to start the video first with the animal just on a constant infusion, because that's the way we typically run things in the operating room.
Then we'll let the controller take over, and we'll move the level of the target level up and down. So let me just play this video here. So right there, the [? spectogram ?] at the bottom. And you can see the lines wandering way above one, because in the second panel there you see the infusion rate is constant.
This is being held like this, but the marker is doing this. It's not controlled. Now what we're going to do is we're going to turn on the controller. The controller comes on.
And now it holds the market right at one. And what we'll do is we'll drop it down to-- we'll take them to an actually-- a deeper level of anesthesia here in just a second. [LIGHT LAUGHTER] The time scale is in the order of minutes there.
So we dropped it down, taking the animal a little bit deeper. And what we'll do is we'll bring it up again. So we can-- up to a higher level, all right? So less unconscious. So it's possible to do this the same way that you would have an autopilot, let's say, guide a plane when you're in mid-flight.
And I just want to point out something here really important. When the system took over, look at this. So this is the animal getting deeper, deeper, deeper, deeper, deeper with just the constant infusion of drug. That's the way the brain is basically taken care of in the operating room right now. We just run constant infusion so people just get deeper and deeper.
Look what the controller did. The first thing the controller did was says, you're way too deep. I'm going to shut off. It actually shuts off for about three minutes, lets the target drift up to where it needs to be, then it turns on controls again.
And look over here, it did the same thing. Over here, the control actually shut off for eight minutes. And then so you could get back to the level where you needed to be. And then sort of reinitiated the control. So this is something-- we did this about nine years ago with rats.
And the FDA told us that in order to test it in humans we'd have to have a large animal model, a non-human primate model. So that's why we've been doing the non-human primate experiments. So what we hope to do is to get this approved for FDA testing in humans. So to date, the FDA has approved no closed-loop system for anesthesia delivery, because they don't trust the systems. We have self-driving cars, right? [LAUGHTER] All right.
And I'm going to be there. And I have to tell you this. So we went down and had a conversation with the FDA. And so they're giving us all these questions, these hypotheticals, because they can always ask more questions than you can answer. So at one point, the guy goes, OK, how are you going to make sure it's cyber secure? I said, I'm not going to hook it to the internet. [LAUGHTER] So one final point, reanimation.
So why not turn the brain back on? And why not use neuroscience principles to figure out how to turn the brain back on? So here's an animal that's anesthetized here, this is work I've been doing with my colleague, Ken Solt, at Mass General Hospital. So this animal is anesthetized with propofol. He has an IV running into his tail vein, and he's out cold. You can see it there. Propofol is white, so that's the white IV you see there.
what's going to happen is, Ken is going to stimulate his ventral tegmental area, all right? Turn the simulation on. Now watch what happens. We didn't turn the anesthesia off.
Turn the stimulation on, and you see he starts breathing like crazy. The anesthesia is still going. And he says, look, I'm out of here, all right? [LAUGHTER] All right, so what's Ken doing? So we're using this very important pathway that starts here in the mid-brain, right here. It's called the meso, mid-brain, cortical pathway.
And this is one of the pathways where Ritalin works, the same Ritalin that we use to treat ADHD. And it releases dopamine. And in fact, you may know this, that there are people who self-administer drugs like Ritalin to improve their-- help keep their minds sharpened.
This is dumb, do not do this, all right? [LAUGHTER] All right? OK. But that's what I call science from the word on the street. The word on the street says, you should give something to turn the brain back on, so it can be sharper and you can function better. And so that's what we should be doing at the end of the case in anesthesia, and this is different. This is conceptually different from what you do with naloxone when you have a person who's overdosed with an opioid. So here you have the opioid sitting here.
It's bound to the receptor, and naloxone comes in and competes for it, removes it, and you remove the effect of the opioid. This is different. This is really taking advantage of the neuroscience of how the brain architecture is laid out. So what you have is into any given neuron you have inhibitory and excitatory inputs. When you anesthetize someone you have pushed those inhibitory inputs beyond the excitatory inputs.
So why not drive and excitatory pathway to bring the person back? And that's what this is. This Is a very important cognitive pathway coming from the mid-brain going up to the cortex, the mesocortical pathway. It goes to the limbic system there. And what I showed you there was Ken doing this electrically. We've also done it optogenetically.
So this is something, what we're studying, not only for the purposes of waking folks up from anesthesia with drugs like Ritalin, but also for waking patients up from coma. We have a trial of this going on right now at Mass General Hospital doing this. So four ways we can use science and technology to improve anesthesia care for the elderly, intelligent monitoring, multimodal use of drugs, closed-loop control, and waking the brain up after anesthesia.
All very doable within the next three to five years. Nothing up there is out of reach. And one of the things that we're doing is we're putting together a center to take on this and other problems in a very focused way, kind of what Rafael mentioned. So the curiosity of MIT, taking on problems that need to be solved, and this is one of them. And going beyond just anesthesia, but things that relate to anesthesia, improving anesthesia by developing more site-specific techniques. What we should be able to do is just go to a couple, two or three brain regions, and activate them, and control them, and use that as a way to generate anesthesia, not having the drugs go everywhere.
That's why you have all these profound side effects. From anesthesia insights, we have better ways to sleep. I showed you something about the closed-loop control. We even have a nexus with working with Li-Huei. And I have to tell you about this.
Depression, it turns out that there's very good data that suggests that use ECT, electroconvulsive therapy, to treat patients with depression. So to treat them you have to anesthetize them. And then you apply the shock therapy. There's good data to suggest that you didn't have to apply the shock therapy. You can just anesthetize them for a period.
And then just wake them up, all right? More work for anesthesiologists, see? [LIGHT LAUGHTER] All right? I mentioned the wake up here. And as I said, the idea is that we're using it to wake the brain up. We're also using for coma recovery, and it turns out there's a very strong intersection between the way that animals go into profound states of, I'll call it hibernation, and the way-- and the state that people are in when they're under deep anesthesia. And so these are some of the new ideas that we should be exploiting, and that's going to be the focus of our center. So I'll stop there.
Thank you very much for your attention. [APPLAUSE] Whitney Espich: Thanks for joining us and for more information on how to connect with the MIT Alumni Association please visit our website.
2022-06-24 21:19