Sickle Cell Disease: How New Technologies Are Transforming Patient Lives
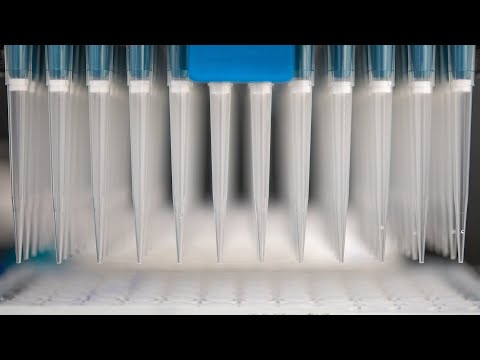
- Hello everyone. Welcome to today's future direction of lab medicine webinar for an update on sickle cell anemia, how new technologies are transforming patient lives. I'm Dr. Bobby Pritt, the division chair for clinical microbiology at Mayo Clinic in Rochester, Minnesota. And our speakers for today's webinar, our Dr. Maggie DiGuardo and Dr.
Justin Juskewitch, both of whom are assistant professors of laboratory medicine and pathology and pathologists within the division of Transfusion medicine at Mayo Clinic in Rochester. So now I will turn things over to our group to begin our presentation. - So good day everyone, and greetings from Mayo Clinic.
It is a privilege for us to be here to discuss with you some of the really exciting changes that are happening on the, in the field of sickle cell anemia and our management and treatment of these patients. Both Dr. Diardo and I have no disclosures to rely on. So today we are going to cover the following learning objectives. We're gonna start by describing the prevalence and the pathophysiology of sickle cell disease, so what it is and why it's important. And then through the lens of a clinical case study, we are going to summarize some of the current clinical management approaches we have for patients with sickle cell disease, particularly the non-curative approaches.
And just as a note, we do have the permission, written permission of the parents of the patient that we're gonna be discussing today. Then I'm gonna hand it over to Dr. DiGuardo who is an expert in both transfusion medicine and cell therapy.
And she's going to focus more on the curative treatment modalities for sickle cell disease, including some of the new FDA approved treatment modalities that are out there and describe how those work and how they potentially are going to change how we manage sickle cell disease in the future. Dr. DiGuardo and I are both transfusion docs. I'd be remiss if we didn't mention a red blood cell somewhere here in our talk. So we are going to talk about sickle cell disease, which is a hemoglobin apathy or a disorder of hemoglobin. Red blood cells are one of the a nucleated cells within our body and their cytoplasm is chockfull of a protein called hemoglobin.
This protein is made up of four polypeptide chains, two of each of two varieties that we'll talk about. Within each of those polypeptide chains, there is a molecule of heme and in the center of that molecule, heme is an iron cation. It's to that iron cation that oxygen binds when it is absorbed through the body in the lungs and then it is unloaded in the tissues that need that oxygen to make energy. Now hemoglobin throughout human development does not stay the same, and so our body undergoes a pre-programmed process of changing hemoglobin during early development.
Now, regardless of what phase of development, two of those four polypeptide chains that help make up hemoglobin will always be alpha chains. But those other two polypeptides undergo a set of programmed changes from fetal development to adult development. And those genes, whether it's the gamma gene, delta gene or beta gene, all live together in a very small region of chromosome 16 and they're regulated by a single regulatory apparatus that helps facilitate that change.
So in utero, the developing fetus will predominantly have fetal hemoglobin or hemoglobin F, which is made up of two alpha chain polypeptide chains and two gamma polypeptide chains after delivery and during early neonatal development, there is this switch from fetal hemoglobin or hemoglobin F to over the subsequent several months, predominantly hemoglobin A or adult hemoglobin, which is made up of two alpha chains and then two beta polypeptide chains. There is also a small percentage of adult hemoglobin that is not made up of hemoglobin A, but is made up of a variant called hemoglobin A two, which is made up of two alpha chains and two delta chains. But this whole process of switching from fetal hemoglobin to then hemoglobin A and A two is all controlled by gene locus on chromosome 16. Now, sickle cell disease is caused by an inherited chain change to the hemoglobin, a form of hemoglobin.
As I mentioned, hemoglobin A is made up of two alpha chains and to beta chains. Individuals though can inherit a copy of that beta chain that contains a single nucleotide chains change from adenosine to thymine within codon six of the Beta GLO globin chain that causes a single amino acid change in the sequence of that beta polypeptide going from a non, sorry, a negatively charged polar amino acid glutamate to a non-polar non charged amino acid valine. And we call the variant that contains this valine in position six. The hemoglobin S variant that hemoglobin S variant creates a non a sticky non-polar amino acid on the surface of hemoglobin S.
And so if one inherits two copies of hemoglobin S one then has only hemoglobin S within one's red cells. And what happens is when oxygen is offloaded from the hemoglobin S out in the tissues in that deoxygenated state state, those hemoglobin S molecules will actually polymerize because of those sticky residues and they'll create these inflexible filaments within the red blood cell that cause the red blood cell to actually morph shape from its typical discoid shape to this sickled shape. Now early in the lifespan of the red blood cell, this will cycle back and forth as the oxygen is offloaded.
These filaments will form, they will make, they will make these sickled shape red cells out in the small capillaries in the tissue, and then when they get oxygenated again, they'll flip back to their discoid shape. But over time, this back and forth morphology change will eventually become irreversible as the red cell ages. And so as a result you will end up with sickled cells even in your circulation. Even in the oxygenate state, these sickled cells are problematic because they have a hard time traversing the small capillaries out in the tissue and they cause these microvascular occlusions and ischemia on the tissues. And this happens again and again and again and again during the, the life of individuals with sickle cell disease. As a result, it's that ischemia on the tissues that causes all the disease manifestations that I'll mention in a minute.
I would be remiss not to mention that you don't just have to have two copies of this hemoglobin S variant in order to manifest sickle cell disease. There are individuals who inherit different variants in in their other beta chain in which they can inherit a single hemoglobin S and then a deletion of the the other beta globin chain or a hemoglobin C variant or some others and they all will manifest with sickle cell disease. But having one copy of hemoglobin S and then one canonical hemoglobin, a variant inherited from your other family, me or from your other parent, actually doesn't manifest in disease.
Having that hemoglobin A mixed in with hemoglobin S prevents that polymerization when the oxygen is offloaded. We call that state sickle cell trait, and almost all individuals with sickle cell trait will have no symptoms. The one of the problems with hemoglobin S and the sickling of the red cells is it causes the viscosity of affected individuals blood to increase and the magic number, the magic percentage of red blood cells in the circulation or the hematocrit for individuals with sickle cell disease is 30% beyond a hematocrit of 30%, 30% red blood cells. As of their representing their total blood volume, the blood viscosity goes up exponentially. And so this is a key e value that we try to maintain individuals with sickle cell disease in in order to make sure that they don't have sequelae from their sickle cell disease and this increased viscosity.
You also see a similar phenomenon when a talk, when you look at the ability of hemoglobin S to offload oxygen to the tissues, that efficiency of oxygen delivery also falls off starting at a hematocrit of 30% or greater or hemoglobin of 10. It is these sickled red cells that cause these microvascular occlusions on and off throughout a patient's life that cause the wide spectrum of symptoms that we see in sickle cell disease. That's some of these manifestations are more common in individuals sickle cell disease, some of them are more rare. Some of the more common ones that individuals with sickle cell disease will experience during their lifetimes are strokes or occlusions of the vessels within their brain leading to death of brain tissue and and permanent deficits.
We also frequently see pain crises. These are caused by occlusion of the vessels without in the periphery and that ischemia is incredibly painful as those those affected tissues are starving for oxygen. We can also see similar occlusions within the small vessels of the lungs that can cause a phenomenon called acute chest syndrome. And then over time in the spleen, which is the organ that is responsible for filtering blood, these repeated occlusions in the small vasculature of the spleen can eventually cause the spleen to fibrosis and shrink and by adulthood, individuals with sickle cell disease functionally have no spleen. During that process, though during childhood, it's not uncommon for these occlusions to co block the flow of blood throughout the spleen and you get these episodes of splenic sequestration.
While these are the most common disease entities or disease manifestations, sickle cell disease, almost every organ can be affected is is shown in this slide because the occlusions that occur happen throughout the body. So sickle cell disease is incredibly frequent in some regions of the world. And in fact, at any given moment in time, there are millions of people worldwide affected by this disease process. It is particularly common in Central America, south America, Sub-Saharan Africa, the Middle East and the Indian sub continent. And in fact, it's so frequent that if you look at the frequency of those beta globin alleles within those populations, 25% of the alleles being passed on to children in these areas will be this hemoglobin S variant in Africa, in the Middle East, in the Mediterranean, in India. Given how devastating sickle cell disease is in affected individuals, why then is this gene constantly paying passed on in some areas of the world? And the belief as to why this variant is so frequent actually comes back to Dr.
Pritz a field of expertise which is parasitology. So in these regions of the world, there is a parasite called malaria that infects red blood cells that is transmitted by mosquitoes endemic to these regions. In these areas where malaria is endemic, if individuals inherit one copy of the hemoglobin S gene and then a normal copy from their other parent parent, they are actually protected against mal infection. They have less severe disease because individuals who have that one hemoglobin S variant while actually have 50 to 90% fewer parasites and they become infected malaria compared to someone who doesn't have that single hemoglobin S gene or allele.
And as a result, it is thought that the sickle cell allele is actually protective against malaria infection, but in its homozygous state causes severe disease. So I'm gonna cover through a case study of a patient that we've been managing here at Mayo Clinic in Rochester, Minnesota, some of the non-curative kind of standard treatment approaches for those with sickle cell disease and kind of their rationale for use. So I want to tell you the story about a a girl who was four years old when she first presented at our here at Mayo Clinic Rochester. She was from another part of the United States, moved here at the age of four with her parents.
She was diagnosed with sickle cell disease thanks to neonatal screening soon after birth. And when she arrived at our doorstep at the age of four, she really hadn't had any of the major common sequelae of sickle cell disease yet she hadn't had a stroke, she really hadn't had any of these recurrent pain crises or acute chest syndrome or splenic sequestration. The only thing she had had related to her sickle cell disease was a history of urinary tract infections and kidney infections at the age of two that were treated at this point in her care, she was being managed with a medicine called hydroxyurea. And the reason hydroxyurea is a mainstay for sickle cell treatment is the following, and it actually comes back to that chromosome six complex that I told you about that manages the switch from the fetal hemoglobin with the gamma chain to then the beta and delta chains that make up hemoglobin A and hemoglobin A two. It's there are individuals who actually have an inherited a copy of the beta globin locus and chromosome 16 that actually have a mutation within the regulatory apparatus of that locus. And it actually causes them to not flip from hemoglobin F to hemoglobin A as they e exit the neonatal phase of development and go into adulthood.
These individuals that they inherit, one allele that has this mutated regulatory complex will actually have persistent hereditary persistence of fetal hemoglobin, which means that they will continue to have fetal hemoglobin levels of 20 to 30% throughout their adulthood. It was discovered in very rare individuals out there who inherited a hemoglobin S variant from one parent and an HPFH variant from the other parent that these individuals, because they have persistent expression of hemoglobin F thanks to that inherited HPFH allele, that even though they have an hemoglobin S variant on their other allele, they have virtually no features of sickle cell disease. And it's thought because having that 20 to 30% of hemoglobin F within the red cell mixed in with hemoglobin S prevents the polymerization of those filaments. No polymerization, no sickle cells, no sickle cells, no disease manifestation.
And so hydroxyurea was first discovered post civil war and has been used since the 1960s in as one treatment for myeloproliferative diseases because it interrupts DNA replication within the bone marrow as these white cells are being produced. It was also found in those individuals who have already made the flip from hemoglobin A to hemoglobin A starting at like nine months, that hydroxy urea would actually cause the mar bone marrow to keep hemoglobin F production on to these like 20, 30% levels. And as a result is protective against some of the the disease manifestations of sickle cell disease.
So the famous trial is a mesh trial which was published in the mid 1990s. Individuals were randomized to take hydroxyurea or no hydroxyurea who had sickle cell disease. And it was found, it statistically significantly reduced the number of pain crises and achi acute chest syndrome episodes in patients on hydroxyurea. And in fact, the benefit was so profound during the clinical trial that in the middle of the trial they stopped the trial because it was believed to be unethical to continue with the control arm because of the profound benefits seen in these patients. So that's why hydroxyurea is a mainstay of treatment and that's what she was on when she entered our catchment here at Mayo Clinic.
But then one day something catastrophic happened to this 4-year-old, her parents were giving her a bath and all of a sudden she's developed these bilateral arm tremors. And it happened once and then twice and then three times by the time they reached our local emergency department on exam in the emergency department, she was unable to control the movements of the left side of her body. She had left upper arm weakness and so on, imaging CT angiogram, which is imaging to look to see if the vessels are still perfusing or still patent heading into the brain. It was discovered that she had clotted off her right internal carotid artery, one of the main arteries into the brain.
And on this special diffuse weighted imaging that's done via A MRI, this particular imaging modality is exquisitely sensitive to areas of tissue ischemia, areas of the brain that are not getting perfused with blood. And as you see on the right side, as labeled on this image on the right side of her brain, there were large areas that of her brain that were not getting oxygen because of this thrombus. So she was having her first stroke. And so we were consulted here in the division of transfusion medicine in the middle of the night to see if we could do an emergency red blood cell exchange as part of her treatment for this acute stroke. So red blood cell exchange is one of the apheresis techniques that are performed across the country to help treat various disease processes. Apheresis means to separate, and this is a process by which we stay hook up the patients to an apheresis machine and in real time separate their blood into the constituent components based on how heavy each component is, red cells being the heaviest and then white cells then platelets, then plasma, and then while in real time centrifuging a portion of their blood, we can remove the blood compartment that is causing disease and then return the rest of the components back to the patient.
And in some cases, like in red cell exchange with replacement of the red cells with red cells that are not affected. So in her case we were removing her sickled red cells, replacing them with donor red cells that did not have hemoglobin S as part of her treatment for stroke. Stroke is considered acute stroke.
So emergency red cell exchange in those with sickle cell disease is considered by our American society for apheresis, a category one first line therapy go in the middle of night treatment. And so that's what we did. We used a device called a spectra optia because she was so small, she did not have enough blood volume to safely fill the circuit of the machine with her own blood. So we had to use a donated red cell unit to fill that circuit, which was about 200 millimeters.
And then in these procedures we aim to at the end of the procedure, have a final hematocrit red cell mass of 30% of their total blood volume. And then we aim to replace 70% of the patient's own red cells with donated red cells. So 30% fraction cells remaining. And as you can see here on the left side of this bar graph, we went from hematocrit of 22% to acrd of just above 30%, which was our goal. And then the sickle crit, the percentage of her hemoglobin and her red cells that was hemoglobin S, went down from eight just under 80% to about 20% with that 70% relative replacement.
The question then is once you have an event like this, how do you manage them going forward to prevent future events? And there are two other key trials I wanna mention that support long-term transfusion support, chronic transfusion support in patients with sickle cell disease. So the first trial was the stop trial. It looked at 130 kids who were affected by sickle cell disease who not yet had a stroke but had vascular changes in their brains based off of doppler that put them at high risk for stroke. And they were randomized to either red cell transfusions to try to prevent their own bone marrow from triggering their production of their own red cells.
So to keep that sickle cripple of 30% or no transfusion support. And what was discovered during this trial was those in the transfusion group had a 30 93% lower stroke rate than those who were in the, the trans in the control group. And again, halfway through the trial it was felt that it was unethical to continue because of the profound benefit transfusions were providing. And so the trial was HAL halted early.
The follow up question then was, okay, you get people locked into long-term transfusion support to try to suppress their own production of sickled red cells. When can you stop? And so this stop two trial looked at about 80 kiddos who had received 30 months of that chronic transfusion support to the point that the vascular changes within their brain had actually reversed thanks to those 30 months of suppression and treatment. And the question was, well now that they've reverted, can you stop or do you need to continue? And so these 80 individuals were randomized to one of those two arms and it, over the course of the trial, two of the individuals in the stop transfusion arm had strokes. Another 14 had reversion of their brain vasculature back to that abnormal state during the trial, but none in the transfusion group.
And again, the benefit was so profound for maintaining transfusion support that the trial was halted early because it was unethical to continue to withhold transfusion support from those randomized to it. So it's the basis of this that we then started a chronic transfusion program in this 4-year-old girl post-stroke and the goal was to keep her hematocrit, her total red blood cell mass relative to her blood volume to 30% to keep her suppressed and hopefully to keep her sickle crib below 30%. As you can see here, the dashed arrow was the red cell exchange in the middle of the night. This was her ongoing red cell transfusions in the outpatient setting. Every four to six weeks, we were able to roughly keep her hematocrit near 30%.
But this strategy did not keep her sickle crit under that 50%, under 30% goal, much less under 50%, which is the red line here. So it was not keeping her bone marrow adequately suppressed. The also the problem with giving just packed red blood cells every few months is each of those contains about 200 milligrams of iron.
And iron can be toxic to the human body in excess. And the way we manage that normally is we control how much our gut absorbs of iron, but we have no way of getting rid of excess iron. So we control it at the point of absorption. But if you're giving transfusions that contain 200 milligrams of iron each time, there's no way for the body to clear that. And so her iron stores continue to to increase or rise.
Over the course of this treatment, she ended up with iron over moderate iron overload. This is very common in individuals with sickle cell disease who are managed with chronic transfusions. And so they have to go on iron chelation therapy and medicines to try to bind and remove the iron. But these medications have extreme side effects. They are poorly tolerated and individuals just feel horrible on these medications. So the Hema service came back to us and said, we can't keep her controlled with just these simple transfusions.
Is there a better way you controlled her really well with that middle of the night red cell exchange? Can we do this long term for her? And so chronic not acute red blood cell exchange is a category one in indication first line therapy for individuals with sickle cell disease to help prevent future stroke. And it's a second line therapy category two indication for those with sickle cell disease who have recurrent pain crises. But this process is not without challenges. There are quite a few hurdles to get someone plugged into a chronic red cell exchange program. The first being that we have to have long-term vascular access and this long-term vascular access isn't just sticking a needle in their arm each time we do the exchange because of their vascular changes to their sickle cell disease. So oftentimes these individuals have to have long-term dialysis catheters in PA place, which it really hinders a lot of their quality of life.
Also, given how small she was, we couldn't just use her own blood to fill the circuit of the unit each time. And we didn't want to continue using packed red blood cells because they have a hematocrit of 60%. And when you turn on that machine that 60% hematocrit is going into her circulation.
And we were worried that that transient he concentration might spike her the viscosity in her blood and cause her to have a sickle cell event. And so instead we developed a process by which we created special reconstituted RBCs within our labs that were at that 30% hematocrit so that we could prime the circuit with something that would be safer for her. Individuals with sickle cell disease also have another challenge when it comes to transfusion support because they're pro-inflammatory state, and because of the number of transfusions they need to help suppress their bone marrow production of these sickle cells, they are at high risk. They are one of the highest risk groups for developing red cell antibodies. So their immune systems recognize something foreign on those donated red cells and they form an antibody to clear them out. They are at six times the rate of developing these antibodies compared to the general population.
Even when you account for a number of transfusions and in individuals with sickle cell disease, if you transfuse an antigen positive unit for which that patient has already developed an antibody, not only do they clear out those red cells, but there is a rare but potentially catastrophic phenomenon in sickle cell disease in which they can have hyper hemolysis in which they destroy their, not not only the transfused red cells that you gave them, but their own red cells as well. And this can be fatal. So this is something we avoid by trying to provide antigen match units, which can be a challenge when you have a predominantly African American patient population and a Caucasian donor population in the United States in which those antigen frequencies vary. And then the other thing is how frequently do you do them and try to time the cadence of these red cells for each individual patient.
We try to aim for a final hematocrit above 30% in order to keep them suppressed until the next one. But we don't want their sickle CCR percentage of hemoglobin S to rise too high between sessions. So for her, we got her a dialysis catheter, which stuck out of her neck, and we did these procedures in the PICU and we figured out a good cadence for her to do these red cell exchanges. And for her it was every five weeks you can see can get her up above 30% hematocrit. And we could keep her suppressed for those five to six weeks. And we were able to keep at a five week cadence, her maximal sickle CRI, right before we do the next procedure to around 40%, which for her and her family was a frequency that worked.
But to emphasize this is not a cure and this creates a significant burden on patients and families. And as such creates a lot of challenges for quality of life. So now I want to turn it over to Dr. Diardo, who's my colleague here in the division of transfusion medicine, who is also a cell therapy expert to talk about some of the curative PLA platforms that are coming online for the treatment of sickle cell cell disease, Dr. DeGrado.
- Thanks Dr. Jessic. You know I was a part of this or I had the privilege of being a part of this with you, but every time I hear you talk about that case study, it gives me chills. So with the, excuse me.
So with the tools at hand, what, what Dr. Jessica, which has been able to do with this patient in collaboration, of course with the clinical team is, is really excellent. However, it does sound like or not sound like, but there are some new options on the horizon here that may actually be curative. So I know the next, I know the title slide says New therapeutic options.
I'm actually gonna take a step back and just talk about some of the options that exist outside of hydroxyurea and re red cell exchange in order to paint a picture for the evolution of how we got to where we are now. One of the previous, or excuse me, one curative option that actually is available and has been for a number of years, started in early 1980s was stem cell transplant. So these were allogeneic hematopoietic stem cell transplants. I think the earliest was done around 1981.
So these are no small feat and require an en an enormous amount of work both for the patient as well as the donor and the clinicians. So we're gonna start with just in terms of the allogeneic stem cell transplants, alle meaning that these are coming, these hematopoietic stem cells are coming from a donor, right? And so that entails an enormous amount for the donor donor selection, carefully and manually matched. And then donor eligibility, so infectious disease testing, screening, and then donor collection, right? So we have to draw those CD 34 stem cells out to the periphery and then we have to collect them, excuse me, on the apheresis machine as well. This also entails patient conditioning, right? So while we're just selecting the donor and they're going through their testing, we're conditioning the patient and at this time we're getting their bone me, we're essentially wiping out their bone marrow in order to prepare them for the new stem cells that we'll be infusing. So at this time, then the patient will go through their infusion and that's no small feat because after that, until they engraft, they'll potentially be incredibly immunocompromised and at risk for multiple, multiple issues with transplant itself. So all that being said, however, I would like to highlight this review paper.
So it shows over the course of about 25 years, a thousand patients were analyzed. And what you can see here is that the five-year overall survival was approximately 93%. So essentially HLA identical sibling transplantation offers excellent long-term survival. What I wanna highlight about that is that the more words you have in front of transplantation, the more challenging, the more challenging it is to match, right? So stem cell transplants are incredibly effective, right? But they're laden with challenges first.
They're allogeneic, right? And initially they started with HLA identical siblings and not everybody has an HLA identical sibling that's disease free. Now, although we can use haploidentical, which are easier to match, donors can still be incredibly challenging to find. There's the sequelae of transplant itself, which is no small thing. And then access, which is what we're gonna talk about for a number of these gene therapies as well. So I put this table up here and this is really simply only to highlight the dates. So hydroxyurea, you can see as Dr.
Jessica Hoch talked about, has been around for quite a while, but it wasn't until 1998 that it was actually approved by the FDA for use in adults. And it wasn't until 2017 that it was approved for adult for use in peds patients. This hydroxyurea, as we know, increases the fetal hemoglobin. And we'll see that time and time again throughout the next, as we talk about some of these other treatments, it wasn't until 20 years later that L-glutamine, omalizumab and vlo to came on the market.
These were FDA approved all within the same time period, but again, approximately 20 years later. And then now we have the gene therapies, which were approved by the FDA in late December of 2023. So the FDA approves the first gene therapies.
This was done in November, or excuse me in December of 2023 in this country year of needs credit for approving these for use in 2023 of no, or excuse me, November of 2023. So these were huge, right? This was no small feat. And I wanna highlight again the timing.
So these, these are some tweets and as as we go through, I'll just highlight a few of the different comments that came about. So Jennifer Doudna from the Dower lab, she was one of the, she created, or ex, excuse me, helped bring up this CRISPR system and also won the Nobel Prize. Going from the lab to an approved CRISPR therapy in just 11 years is a remarkable achievement.
We also have VJ St. Karen who led the GWAS studies. This is tremendous advances and remarkable to see our discoveries in just 15 years get to where they are. The editor in chief of the CRISPR journals who says that this is momentous for the field. I wanna highlight the fact here that he's talking about highlighting the momentum with regulatory agencies in a global context. And then we have Patrick Sue of one of the early developers of the crisp CRISPR genome editing, working with Bang Zang called it a huge victory for biotechnology patients.
And so I really wanna emphasize the timing, right? And in our world, 11, 15 years is a drop in the bucket. And I also wanna highlight that everybody is talking about this in a global context. But luckily we also, as we have our enthusiasts, we also have our pragmatists. So British lawyer and ethicist, Julian Hitchcock states, will we get it on the NHS some dubious? And then we've got our hematologist que sharma who's asking the really important question here.
Now the real work begins to make this therapy truly accessible to the patients who need it most. So we're gonna touch back on that access point here in just a moment. So what are these therapies? What's all the why? What's the big deal and how do they work? So I'm gonna present a case report really quickly. I'm not gonna go into too much detail, but I'm gonna give you the highlights here for the pre gene therapy. We have a 33-year-old patient. So this was a case report presented in the New England Journal of Medicine in 2021 and pre gene therapy, this is a single patient IND with a 33-year-old female with sickle cell disease.
She's sitting at a hemoglobin of 7.2. That's where she lives for purpose, for reference, you and I, and most of the audience probably sit anywhere between 12 and 15 grams per deciliter. So this is quite low. She has a hemoglobin half of 9.1%.
We're gonna, I want you to paid attention to that. And a hemoglobin S of 74% on average per year, she has approximately seven vaso-occlusive events, approximately four hospitalizations and five red cell transfusions post gene therapy. So this is approximately 15 months follow up.
This patient now has 12, is sitting at a hemoglobin of 12 grams per deciliter, right? Really amazing. A hemoglobin F of 43.2%. Please keep that number in mind. We have a hemoglobin S of 52.3%. We did have 114 AEs adverse events that were detailed throughout the trial.
Most or all of these were considered related to the transplant. Zero vaso-occlusive events. And had she was transfused and independent after day 19, those first 19 days, nobody would be transfusion independent due to their pancytopenia.
We consider that to be day of engraft men. Okay? So what did this, what is this gene therapy that created such remarkable results in this patient in 2021? Well, this was cavy. So this was the crispr, this was Cassy, which is a drug, the gene therapy, that is a product of a, a collaboration between CRISPR therapeutics and Vertex pharmaceuticals. It's a gene editing therapy utilizing the CRISPR Cas nine system. It is the first ever CRISPR based therapy. It's non-viral, very important. And it's ex vivo.
And by ex vivo, that means that it is generated ex vivo and then it is infused into the patient. Okay? What is the CRISPR system? So let's just give some quick definitions to bring everybody. We're gonna calibrate the audience. Apologies, experts in the room. I'm gonna do this in broad strokes. While I know that there's a significant amount more detail that would go into this, but at least let's just talk about what it is.
So CRISPR stands for the clustered regularly interspace palindromic repeats. These are sequences found within the prokaryotic genome. So they're just exactly what they sound like these C certain sections of genetic material that are palindromic and repetitive. Okay? And we found them in the bacterial genome.
cas, what is the cas? So this stands for CRISPR associated protein. This is an endonuclease, okay? This is, so this is capable of generating double stranded breaks. And the double stranded breaks are the important part here, right? Because you can essentially cut out both strands of the genetic material, both alleles we can target, okay? And these, their gen, this, the genetic sequence that encodes for the CAS is actually outside the CRISPR region. And then combined, what was it? What was the bacterial defense system? So this image to the right shows a bacteria being invaded by viral pathogens, okay? Those viral pathogens, we call them bacterial phss. And so what essentially was happening was the bacterial, excuse me, the, the bacterial defense system essentially is a card catalog of genetic snippets from these bacteria phases they've incorporated into their own genome so that upon second exposure, they can have a very quick reaction. I dunno if that reminds anybody of anything that we have, but it's fairly similar to our adaptive immunity.
Okay? So obviously we can see the double stranded DNA and we're gonna show what the CRISPR CAS nine system here can do. Okay? So here we go. We've got our clustered regularly interspace, short palindromic repeats.
We already know that's that what that is, what that means. Here's your CAS nine, this is your endonuclease, that's guide RNA, right? So what we're doing with the CAS nine is we're adding a guide RNA, which takes us to the part of the genome that we wanna target, okay? In this case, they'll find a complimentary sequence that's highlighted right here. The CRISPR CAS nine system will hone in and target that section of the, or that sequence based on the complementary pairing with the guide RNA at that time. This will generate the CAS nine system, or excuse me, yep.
Essentially we will transduce it into cells and once inside it will target this area of the genome and create that double strand of break right there that you can see. Whereas then we can manipulate. So delete, add to right or change variance there. And then the DNA will come back together, repair itself, and now you actually have a new sequence.
So what exactly is Cavy doing and what is it using for that? How is it using the CRISPR cast system? Well, it's going directly. So it's targeting that BCL 11 A gene, BCL 11 A as Dr. Jessica Witch explained, is the gene or the transcription factor that evolves from the, or excuse me, that comes from the gene that is responsible for decreasing for that flip that he talked about, right? So that flip at birth or in the neonatal period when your GAM globin starts to decrease, your beta globin change start to increase, you go from a fetal hemoglobin state to a hemoglobin a state, right? And so hemoglobin A being your healthy adult hemoglobin, so that BCL 11, a transcription factor is responsible for the decrease in that GAM globin. So once that's turned on, that's when the gam globin starts to drop and your fetal hemoglobin starts to change over. So within the BCL 11 A gene, you can see we've got four exons.
Those are coating regions. And right there in the middle is a erythroid specific enhancer by targeting that erythroid specific enhancer. And this is really just a kind of watering down that CRISPR cast system, but you can see the two scissors on either side. Essentially if the, with the double strand of breaks and the changes that they made to the genetic material, right at that area, they were able to turn off the BCL 11 A gene. By turning that off, that means that transcription factor is no longer being generated and essentially ex, excuse me, specifically for our erythrocytes. And so the gamma globin now can be, can increase, right? And that's flipped. That switch can be flipped again.
So this was really exciting, right? Because essentially they've targeted the fetal hemoglobin, which allows our patients to come up. As we saw, she ended up shows, I think at 11% and ended up at 43% fetal hemoglobin, which essentially made her disease free because we were able to mimic that hereditary persistence of the fetal hemoglobin phenotype, right? And so that's why this patient, we hadn't changed anything about the hemoglobin A, we didn't target the, the actual sickle cell, the mutation that causes the sickle cell disease. What they did was they targeted the fetal hemoglobin and brilliantly. So that's the cavy. How does L genia work? Because this was the second one that was also approved by the FDA at the end of 2023. This is a product of Bluebird bio.
This was granted priority review in June of 2023. And it received GSD, excuse me, FDA approval just six months later in December, right? So these are fast tracked, and by fast I mean rapid acceleration. This is via the gene delivery of a lengthy viral vector. So this is drastically different than what we're looking at with CRISPR Cas nine.
And this viral vector did target the hemoglobin, the modified beta globin gene in order to generate a new hemoglobin, a isoform. That's the T eight seven Q. This is also manufactured ex vivo. So essentially L genia mechanism of action. So the L genia mechanism of action, essentially we're gonna modify the betaglobin gene, we're gonna add it to a lentivirus, and we're gonna generate what's gonna now be a viral vector.
This viral vector now is going to be delivered into the patient's CD 34 positive stem cells. And then these are going to then be infused back into the patient. So how does this work? But essentially we take our lentiviral vector, right? It's a vector now because it's carrying modified genetic information. And we're going to take our vector and we're going to take our CD 34 positive stem cells, and we're going to combine them. So this virus will hone, will enter the CD 34 cells, hone to the nucleus, and it will release its contents where then they will then be incorporated into the host genome, the host therefore being that CD 34 positive stem cell. And once it's in that genome, then we will take those CD 34 positive stem cells, expand them and infuse them back into the patient because now they're carrying that modified beta globin gene, the beta globin gene that will now generate healthy hemoglobin A.
So where do we get those CD 34 stem cells? Again, this is really to highlight what these patients go through. It's really no small thing. We're gonna use those apheresis procedures. Again, just like Dr. Jessica,
which described they're gonna go through multiple red cell exchanges, bring them up to a healthy level, we're gonna mobilize them, meaning we're gonna give them drugs to pull those CD 34 stem cells after the periphery, and then we're gonna collect them. Those cells are sent to the processing facility where they'll undergo that, that modification, like I just mentioned with the viral vector. They'll be so genetically modified and expanded into enough cells to make a healthy dose and they'll be returned to the treatment facility whereby they will then be infused into the patient.
So why, so these are, so obviously what I've been able to show is all of the excellent aspects of these gene therapies. And in very brief description, I'm not doing them justice, I'm acutely aware of that. But some of the mechanism of action just to really, I think it's important because I think we're gonna see a lot more of these coming around. And so to understand the difference, especially in healthcare, what you're gonna be delivering to your patients. But what's the data supporting these just outside the fact that that patient did really well in that 21 20 21 case report? Well, they were both single arm multicenter trials. They both included adults and adolescents.
The inclusion criteria was fairly similar for both, maybe perhaps just a bit more stringent for casca. The primary outcomes were essentially the same as well. And we looked at 44 patients total for cavy 32 for L Genia. Really though we were only able to not, we had nothing to do with this, excuse me, but CAS CRISPR Therapeutics was only able to follow up with 31 of those 44 patients.
So the numbers really came out to be similar. And you can see that 93, 90 4% and 88% achieved the outcomes that they were looking for. So really, really successful. Right? And there's quite a bit more data to show. I know people have questions about off start, off target integration with the lentiviral vectors and whatnot, and all of that was really well ex explained and detailed in in the actual publications.
I would be remiss, however, if I don't go through at least part of the GENIA outcome, and this is in fact due to or exactly what people were concerned about, we using a lentiviral vector, and that's offsite insertion, right off target insertion that could lead to oncogenic potential. So the L GENIA trial, just for purposes to be transparent, did have the very first two patients did develop both acute AMLs, right? One was MDS that progressed. This was, the trial was stopped for quite a while and these were investigated.
There was the, they looked at the CD 34 blast cell population in these patients, and there was no viral vector in patient two, although they did see it in patient one, where they found it was in the vamp four gene was, which was not considered to be. Essentially, I had no known role in oncogenesis. All I will say about this is that there was an extensive investigation and it was considered part of the natural progression of their disease. Sickle cell patients are 3.6 to four times more likely to develop an MDS or an A ML due to their disease state than the general population.
So this was not necessarily a surprise. However, your first two patients, not ideal. These were published in a separate publication. So you do have to do a little bit of digging if you want to find it. It is listed however on the package insert. All right, so where are we now? And I'm gonna just take another two minutes and I'll finish up here.
So in case you all have questions, but what's the current state of access? So we've talked about what was available, we've talked about what is available, and we've described these gene therapies. And for the most part, what I've picture, what I painted is this picture of their curative. And I think at least with the amount of follow-up that we've had so far, two to two and a half years, we can say that at this moment they are curative and seem to pose very nominal risk compared to the disease itself. Okay? But let's be honest, right? We gotta talk about access. Casca is about two to two and a half million dollars per patient.
And l Genia is approximately 3 million per patient. There's likely only available at larger medical centers. Centers must also be approved as an authorized treatment center. So that's an application and you have to be granted that access. But on the upside, the commercial insurers, blue Cross Blue Shield are planning to cover both.
So that's excellent. How about our Medicare Medicaid patients though? Let's talk about them. Currently they are not being covered. Okay, but that is not all doom and gloom because CMS in a somewhat unprecedented, I believe, although I don't, I will not, I cannot claim to be an expert on CMS or this aspect of the, of the work. But they have created their CGT access model.
This is the cell and gene therapy access model. They have recognized that these cell and gene therapies play a PI could potentially have a pivotal role in curing patients with rare diseases who are on Medicaid and who would not otherwise have access to them. Given this, they've laid out this plan, which I believe is fairly novel for CMS, and they'll be negotiating with man with the manufacturer. So Lyft Genia and, or excuse me, Bluebird Bio and CRISPR Therapeutics on behalf of the states to make this available through the state Medicare agencies by 2025.
Next slide please. This will be an outcomes based agreement that CMS will negotiate, right? Then the states will have the potential to enroll. This was actually all just accomplished, I believe in August. So March and August were two different enrollment periods. And then after that, once they've established who will be a part of it, CMS will have a large role and help with the implementation and then the management of this.
So really unprecedented for CMS to do this. So, and this is such that each state does not have to individually negotiate in order to figure out a new system. So it's really excellent. And also the goal here is to roll this out in January of 2025.
Anybody who's interested in more of that conversation, there is a webinar. I know I'm not, this isn't, I do not work for CMS, but if you're interested for more of this information, especially for our own practice, they are providing on cms.gov a webinar just to detailing this, I think, and doing it more justice than I am right now. All right. What are we doing here?
Sorry, Florida's pursuing it and they're an active authorized treatment center preparing to bring it online. Mayo Clinic, Rochester, we're waiting because we have to wait because of the Medicare, Medicaid and Arizona, they will not have any plans to bring it online due to patient population. But they have collaborate with the hospital down there quite close by. That is planning to bring it, to make it available. - Well, thank you Dr. Dedo and Dr. Sitz, that was outstanding.
We do have time for, we have a couple minutes for questions. So for those of you who wanna submit questions, we'll, we'll do our best to answer them. Please use the q and a feature. One question is, these genetic therapies have been, have these genetic therapies been tested on other known hereditary or genetic diseases? So it's very exciting what we can do. Yeah, - No, yeah, that's a great question. So there are clinical trials and process.
One that I know, and I didn't mention it here but should have was the beta thalassemia patients. Not only are they being trialed, but they've actually been approved to be used for beta TH patients as well. So there are other ones in the works, but the progress of those, I honestly can't speak to. - Well, that is very exciting. It really, and it's cool.
And yeah. And there was a question just for clarification. So these new treatments, they have been given to patients already. So is that the question? So that's the question. Outside of the study results, presumably - They, so they're available, they're FDA approved and they're available now. So if the hospital system is set up and is filed and is appropriately you deemed appropriate, then they are a A, they are available for patients.
The clinical trials, the University of Minnesota was actually one of the sites for the clinical trials and treated a number of different patients with these gene therapies. So yeah, so outside of the clinical setting, it's a great question. And actually I've not followed up on any, any of patients that have received it since the FDA approval. - Well, thank you Dr. Dedo. We're at the top of the hour, so I think we'll just finish up. And so we have a slide. I'd like to share this.
For those of you who are interested, we have our 2024 classical hematology conference coming up in October. There are in-person and virtual options available. And again, I'd just like to thank our speakers and thank everyone who's participated in today's webinar.
I realize there were some more questions we did not get to. So if your question was not addressed or if you have additional questions, please include them in the evaluation. - Thank you for participating.
Please click the button below to complete the evaluation and obtain credit.
2024-10-13 19:38