A Deep Look into COVID and a New World of Innovation
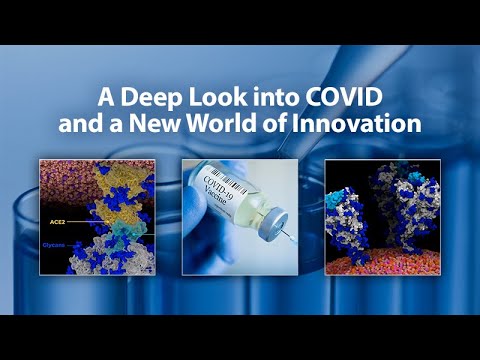
[MUSIC] Welcome. I am Kit Pogliano, Dean of the Division of Biological Sciences at UC San Diego. I am pleased to welcome you to this deep look event which is focused on the many innovations that have resulted from the unprecedented challenges posed by the COVID-19 pandemic.
Today, we will hear from UC San Diego's Chancellor, two outstanding faculty and an alumnus who, like many top scientists worldwide, have focused their creative energies to combating this pandemic. First, it is my pleasure to introduce UC San Diego Chancellor, Pradeep Khosla, who has led the campus since 2012 and who leads the multifaceted response to the pandemic that has allowed us to safely return students to campus, partner with San Diego County and school districts. Chancellor Pradeep K. Khosla is an internationally renowned electrical and computer engineer, recognized for his seminal contributions to secure software and intelligent robot systems. As UC San Diego's Chief Executive Officer, Khosla has positioned the institution to define the future of the public research university by activating the institution's first ever strategic plan and launching the campaign for UC San Diego an ambitious 10 year two billion dollar endeavor and to transforming the university physically, culturally, and intellectually.
Under Khosla's leadership, UC San Diego has expanded college access and affordability for undeserved students, initiated campus-wide interdisciplinary research initiatives to foster collaboration and solve societal challenges and strengthen university and community partnerships to drive regional impact. UC San Diego's response to COVID-19 called the Return to Learn program has made national headlines for its many innovations, ranging from COVID-19 tests and vending machines, hundreds of wastewater testing sites across the campus, and partnerships to launch vaccine super sites. Chancellor Khosla, thank you for joining us here today and thank you for your innovative leadership during these unprecedented times. To start, can you please tell us how the early plans for formulating the Return to Learn strategy came together? Thank you, Dean Pogliano. I really appreciate you inviting me for this conversation. As I think about how do you make plan scheme together, I cannot help but counting the number of months we've been in this pandemic, and it is nearly a year.
I think it was around March 11th or something like that that order out to send the students home. The plans for this started about a month or so before that, I was talking to Chip Schooley who, for people who don't know Chip Schooley, he's one of the leading infectious disease people on our campus. It's also part of the administration dealing with international students.
We were just chit-chatting and he talk about this virus being a potential problem and it could lead us to close the campus completely and shutter operations. That scared me a lot. I mean, I was very concerned by that statement.
The more I thought about it, the more I realized that if we were to get ahead of this, we could actually be operational while keeping our community safe. That's how it all started in that little conversation in my office. What made it possible for UC San Diego to be the first university in the nation to announce such ambitious plans for repopulating university campus as safely as possible? I think what made it possible was my understanding that we had just about every specialty that was needed to address this problem right here on campus, number 1.
We had a very collaborative faculty who would cross boundaries very seamlessly. I just made sure that money and resources were not going to be an impediment. I promised myself early on that we're not going to use money as a reason to not do the right thing. Once I had all of that in my head, I was now a free person to start making bold promises and moves, betting 100 percent or on my faculty to deliver, without even knowing whether they would or not.
But it worked because that's the confidence, the chance that these two have in this faculty. That's great. Why was it important to bring together experts from so many different areas. You still work with people in infectious disease, modeling, bioinformatics, and student affairs, and biologists. Why were all these people important for the response? I have to tell you, it was not clear to me that these people were important for the response upfront.
But the more questions I ask and people who worked with me know that I have this bad habit of asking too many questions, and I do that partly to learn more and partly to force people to think a little bit differently. The more questions I asked, the more I realized that just being an infectious disease person was not going to be good enough. For example, I was talking to Chip and I said, "How do you know how does a virus propagate?" At that time, I remember it was more like a surface propagation model, that's where the infection was staying and traveling. Well, I said, "Okay, I get that. But between me, the human having the virus and touching the surface and somebody else coming and touching the surface, what does a population propagation look like?" Chip says, "You know, we have this great faculty member, a young faculty member whose name is Natasha Martin and she's one of the best modelers." I said, "Okay, let's bring her in."
Then I had this question of, "Okay, it's on a surface. How long does it last on a surface? What do we know about that?" If you just think about me and Chip and others thinking about this as a research problem, we kept on asking questions, and that led to adding more and more and more people to this team. That's great. Thank you. One of the most striking aspects of the campus response is exactly this large team of experts that you brought together that don't typically work together. As a leader, how did you bring these individuals together so productively? Look, I think part of it was luck but I think part of it also was the DNA of this campus which is all about collaboration and problem-solving.
I think part of it was the urgency of the issue and the importance of the issue that everybody felt committed to really address the issue rather than growing their own acts. That's where I think I left out. I did this in a slightly unorthodox way where we would have these meetings on Zoom with 50, 60, 70 people in the meeting.
It was more like a research lab meeting, if I just look back now, and Kit, you are involved in these meetings where everybody could ask questions and answer, and we would have give-and-take, and then in the end, we would have some directional answer which would have refined later on. It worked out really well. Going forward, I'm thinking, maybe this is a better way of organizing solutions to issues where the problem is not defined a front completely where the evolution of the definition keeps on happening over time and the team keeps on changing and morphing over time, adding more people, not adding, deleting some people that [inaudible] stuff, right? In this case, we only added people. Today is a cog meeting. There's like 80 people on the call. Yeah, I've really enjoyed those meetings.
One of the things that's really interesting is the flat structure of people are from all different levels of the institution with all different levels of expertise, and we never know where the productive question will come from. We added people. For example, when everybody was thinking this is surface transmission, our faculty, Dr. Kim Prather and also Dr. Chip Schooley, started talking about no, it doesn't make sense, it is more aerosols, it is more air transmission. Even though the CDC had not applied, the WHO had not applied, my view is my faculty will apply so I'm going to listen to them. I brought Dr. Prather to be part of this conversation.
It only enrich the whole conversation while force people to think slightly differently. The same exactly at one point, we did not know much about air circulation and the issue of air circulation. You can post a question, and then we had people working on air circulation, air models, and modeling the air flows. [LAUGHTER] That's been incredibly informative as well. Thank you so much for your leadership throughout this time.
No, thank you for your leadership. I think without you, the other Deans in the faculty, we would not be where we are. Why was it important to have a foundation of three pillars for Return to Learn? Viral detection, intervention, and risk mitigation. Looking back, it looks like we had solved this upfront. But as I look back and I'm trying to be honest, I think this also evolves as we thought about everything we had done.
We said, now, how do we categorize what we had done into the strategies we were following? Clearly, if you want to manage a pandemic, you've got to detect the virus, you got to understand where is it traveling, how far is it traveling. You've got to figure out how do you stop the travel which is some combination of intervention and risk mitigation. Then you've got to bring all the technologies into play.
We were, for example, the first one back in April when we announce the wastewater testing, nobody knew what it was. Folks, including Dr. Rob Knight said we can do it. My view is if my faculty tell me we can do it, then I'm just going to bet on my faculty and I'm going to double down on those bets.
There we were. Rob Knight actually delivered. [LAUGHTER] Great. [LAUGHTER] Can you please tell us about some of the innovations that allowed Return to Learn success? I think there are many innovations. Clearly, wastewater testing was one of them.
Clearly, it seems obvious now, with the recognition that it was in the air and aerosol transmission, the recognition of that was, I think an innovation, not the aerosol. Does that make sense? I think the recognition that airflow might have some role to play in mitigating the risks and congregate settings. Then at some point where we were going from hardly any capacity 200 to about five or 6,000 a day the innovations which actually people don't talk about, I realized that the federal government at that time could cut off our supply chain anytime they wanted. They were actually doing that. There were hijacking supply chains.
We had four different supply chains established. We had Alumina, we had Thermo Fisher, we had multiple ways of doing this. We had saliva testing, we had nasal swabs.
We had like three or four different supply chains established so that they could not take in all four away. They could take one or two away, which would diminish our capacity, but they could not bring this operation to a halt. Now, it looks like we were not trusting of the bureaucracy that exists. The fact is we were not trusting other bureaucracy that existed then on the outside because they were actually trying to mess with this operation and my goal was to keep my community safe. At one point we went from twice a month testing to once every week testing and we realize that the issue of access was problematic.
If every person had to make a phone call or go to website, it would not work. It would not scale that easily. Being an engineer, and especially an IT person, I know that when you want to scale something fast, you got to bring the friction down to zero.
The best way to bring the friction, the friction in this case was picking up the phone call going on a website, so we did this vending machines. Somebody had this idea and we set up, let's do the vending machines there. Now, we have vending machines nobody schedules anything. You just go swab yourself and there we are. We connected the ability for a soft swabbing test, build the vending machine and put it all together. None of this was the plan a t equal to zero.
All of this evolved over time. It's really good. Do you have a favorite innovation or something you consider the most innovative aspect of Return to Learn? I think there's many innovative aspects of Return to Learn, but I can tell you what, to me, was the most surprising aspect of Return to Learn is a different way to think about this.
When we were thinking about this, the community was saying, wait a second, you're going to bring 10,000 students here and these people are not going to behave properly and they're going to make us all sick and you're just going to hurt the community, which, actually, was very concerning to me, including some of our students, and faculty, and staff signed up for this type of rhetoric. I had to think very deeply about this talk to many people. In the end, we did bring about 10,000 students back and what was most surprising to me, partly because of our strategies, but partly because of their commitment, was the behavior of these students. The single biggest determinant of a system falling apart, whether cybersecurity or pandemic is a human being.
In this case, the human being being our students behave very well. Our Vice Chancellor for Student Affairs, she ran a spectacular operation motivating them to behave well. We had multiple things happening where we had held ambassador program. These were peers who would walk around campus watching for people not following protocol. If you are wearing a mask, you might get a five dollar gift card for Starbucks. If you're not wearing a mask, you would get a little tap on your shoulder saying, please wear a mask.
It was all peer-operated, peer pressure, people working with each other to secure everybody. That was actually an innovative aspect. As we are in this pandemic right now, we have told everybody that if you test once a week for 10 weeks in this quarter, we will donate $50 on your behalf to the food pantry and other needs for the students. This gives them a good motivation to really help their colleagues, their peers who need help, by just behaving better. Guess what? We are seeing a lot of uptake on this.
Fantastic. Looking back, would you have done anything differently? I don't know. I don't think I would, to be honest with you, I know the right answer is I would have, but I have to look back. If I knew the whole problem, this is not the way one would solve a problem, but the more I thought about this, it seems to me that every problem keeps on evolving as we learn more and more. I would create a dynamic solution strategy the way we had here, where the group starts off as a small group, then it keeps on expanding depending on the needs and the issues that arise in the problem statement and our understanding of the problem.
They just get integrated and then we keep on, as they say, build the plane while flying it and it has worked really well. I know it sounds counter-intuitive to build a plane while flying it because it should not work., but in this case, it did work. We solved the problem while the problem was evolving.
Well, you have a virus that is evolving and therefore the response needs to evolve [OVERLAPPING]. Right. Our understanding of the problem is evolving. If you just take a step back and think about, that's exactly how we, as faculty members do our research because that's how we keep on evolving our understanding and the definition and keep on addressing different branches that arise in the problem. That's great. Thank you. Looking ahead with UC San Diego playing such an active role in the region's vaccination strategy. What's your message to those unsure about getting vaccinated for COVID-19 or those frustrated because they can't yet make an appointment? My message is very optimistic.
It is to be patient. I know it's easy for me to say that, but we have been in this for a year now. I think, as I look at the situation right now, by mid April, we should have a lot of vaccine availability. We're already moving from tier 1A to 1B. The websites are becoming better and better. We are starting more capacity.
As we speak right now, our Petco Park was closed for three days because we had no vaccines. Now, we're at a point where it's not our inability to deliver the vaccine, it's our ability to get vaccine delivery. [LAUGHTER]. Once we have more vaccines, I think we'll be in good shape, remark is open now, so I'm very bullish about where we're headed and I think UC San Diego.
For that matter, any public university, an r_1 public university with this capacity should be playing an important role in helping the community become more secure and safe. That's great. Well, thank you. Thank you so much for your leadership and for making UC San Diego the resource and the community that we need to be.
It's really been a pleasure participating in this process just even peripherally, and I feel lucky to have you as chancellor. Thank you Dean Pogliano and I feel fortunate to have you as one of our Deans. Let me just say a big shout-out to our faculty. They were amazing in the way they collaborated, and most of all to our students, I mean, without you, we could not have done it because of you we exist and without you we could not have managed this pandemic. Thank you very much.
Thank you, Chancellor Khosla. Now we'll hear from two of our faculty and one of our alum about their amazing work that they've done on COVID-19. I'm pleased to introduce Biological Sciences Associate Professor Omar Akbari, who will describe a new tool for detecting the SARS-CoV-2 virus based on CRISPR genetic editing technology.
Omar received a BS, MS degree in biotechnology and a Ph.D. In Cell and Molecular Biology from the University of Nevada Reno. He became an assistant professor of Entomology and the Center for Infectious disease vector research at UC Riverside. In fall of 2017, he joined the UC San Diego faculty as an assistant professor in the section of Cell and Developmental Biology, and 2018, he co-founded and became a scientific advisor for aggregating, a biotechnology start-up company located in San Diego California. Welcome Omar. Thank you so much for having me here today.
I thank the Division of Biological Sciences for hosting this event today. Today I want to talk to you about development of a CRISPR Cas or x based diagnostic system, which we termed sensor. SARS -CoV-2. We all know about SARS-CoV-2. It's the causative virus of a respiratory illness known as COVID-19, originating from Wu Han, China in 2019.
This virus has spread globally, primarily by contact, droplets in the airborne transmission. Since January of 2020, just last year, we have over 100 million global cases and two million global deaths. Those numbers are rising every single day. It's really staggering. Our lab traditionally develops technologies to combat human pathogens. We focus a lot of our work on arboviruses, which are things transmitted by mosquitoes, for example, Malaria, Dengue, West Nile, Yellow fever, Zika virus, etc.
As of March of 2020, our lab pivoted some of our work to working on SARS-CoV-2. The green arrows pictured here are actually people in the lab that stops some of their previous work to transition to working on SARS-CoV-2. This is the last lab picture that we took in our lab since the pandemic started.
When we started thinking about SARS- CoV-2, we identified two main areas of focus. One of them was, there was a need to develop rapid scalable diagnostics at that time. Then there was also a big need to develop rapidly scalable vaccines. Obviously, manufacturing, deployment and distribution of those vaccines. Now the manufacturing, deployment and distribution of vaccines is still a problem today. There's almost eight billion people on Earth.
Some of these vaccines require multiple doses of getting 16 billion doses out. There is in manufacturing problem at this point. We all know that the virus is evolving and mutating and there's new variants. In the future, we may need new vaccines.
There might be second does is in third doses. I hope not, but that's where we're headed. Going back to rapid scalable diagnostics, we decided to focus our work on that.
We looked into this. Really there is three main things to measure for diagnostics. The first thing one, can measure is antigens.
There's antigen testing, which essentially is developing amino acids that can detect the presence of specific viral proteins. When you get infected with the virus, it's going to replicate, it's going to have proteins that you can detect with these antigen tests. These are pretty rapid. They can be portable, they can require minimal equipment. Some of the cons with these, or they require the virus to mature.
They're not useful for identifying asymptomatic or pre-symptomatic patients. As of today, there's actually 14 different approved, FDA-approved emergency use authorization tests on the market. We can also use antibody testing. Antibodies are essentially proteins that the body is producing in response to infections. The pros of these, are they can be used to determine if your previously infected. The cons are, they don't rule out an active infection.
Antibodies can be produced even if you have no symptoms. These also take time to be made. One to three weeks following an infection. As of today, there's actually 69 different FDA-approved emergency use authorization antibody tests on the market for SARS-CoV-2.
Then the gold standard really is nucleic acid testing and primarily RT-PCR, reverse transcription-polymerase chain reaction. This is extremely sensitive. It's easy to program so you can design it the target different regions of the virus. It's very reliable. The cons are that it requires very specialized equipment. The turnaround time takes a bit longer or 10-15 hours.
It also requires a laboratory and trained technicians. As of today, there's 209 different FDA emergency use authorization approved tests for nucleic acid testing. Not all of them are RT-PCR base, but there's 209 total for nucleic acid testing. Another type of nucleic acid test that is being developed, a newer type are these CRISPRDx systems. On the right here, this image on the right, you can see that there's a number of different tests that had been developed. Many of them were developed in 2020.
These use different CRISPR, either nucleases, things that target DNA like Cas9, dCas9 or Cas4 or Cas4b, or even RNA targeting ribonuclease is like Cas13a, Cas13b, and Cas13d. In terms of CRISPR diagnostics, there's two tests that are FDA emergency use authorization for SARS-CoV-2 on the market today. Those are marketed by two companies, primarily Sherlock and Mammoth Biosciences. These tests are pretty rapid, and they can be specific, they can have multiple different readouts. Some of the cons of these is that, they currently require multiple transfers that can be complex to set up and they are not as sensitive as RT-PCR.
We actually developed a CRISPR based diagnostic system, which we call sensor, which is pictured here. I'll talk about that. At the time we were working with this CRISPR ribonuclei called CasRx. It's a CAS13d based ribonuclease that was discovered in 2018.
We were testing the system in animals and specifically in flies. This is a picture of our paper that was published in the CRISPR journal when we tested this system. But basically what we found was that we can get really high rates of on-target cleavage of RNA, but we also get really high rates of collateral off targeting. It was very severe and that we could actually kill flies with this system.
We knew how well the system worked, and so we want the pivot that system to a diagnostic. That's what we develop sensor, which is a sensitive enzymatic nucleic acid sequence recognition system. We started this project of using CasRx to detect SARS-CoV-2 and just March of 2020. By October of 2020, we had our first paper out and made an archive of how our system works to detect SARS-CoV-2 the sensor system.
How does our system work? At this point, it's a two-step reaction where one takes a sample, it could be a swab or it could be a saliva sample, and isolates the RNA and then puts it into this reaction, which is called an RT-RPA, RPA stands for recombinase polymerase amplification. This basically converts the viral RNA into DNA with a T7 promoter, that then goes into an in vitro transcription reaction and also gets detected at the same time. There's two different readouts, you can use either fluorescence or lateral flow. Both of them work pretty well. The whole system takes about 15-60 minutes to complete the assay.
It has a comparable sensitivity to other CRISPR diagnostic systems with a limit of detection of 50-100 copies per microliter, which is good enough for detection of people that are infectious. This is just some of the data that we had from UCSD. This is actual patient's sample data where we tested the two different formats, either the fluorescent assay, or the lateral flow assay on the bottom here, and depending on the viral titer, so this line here is a CT value, the lower the CT value, the higher the viral titer. When you have a really high viral titer our assay worked really well, but as a viral thyristor tapered off, it wasn't able to detect as well. All 36 of these fake patient samples are positive for SARS-CoV-2.
We had a pretty good positive selection, and we didn't have very many false positives, which is great. But the system is definitely not as sensitive as RT-PCR. But the lateral flow format makes it really robust and cheap for point-of-care testing of nucleic acids.
We're really excited about this, the system works pretty well for detecting SARS-CoV-2, and we're working to optimize it a little bit more. This was our first go at this. Some of the future directions of this are that we are working right now to improve sensitivity. Instead of using RT-RPA, we're trying LAMP, which is 10x more sensitive than our system. We're also trying to design it to have less transfers.
We want to have a one-pot reaction where we can just put everything in one tube, and then get a readout. We're also trying to change the temperatures that things work out. We're trying different enzymes and things like that. We're actually doing quite a lot of innovation in terms of diagnostics, which can be useful for SARS-CoV-2 potentially now or maybe the next pandemic or detecting other pathogens. Going forward, I recently pitched this deck to DARPA, and the idea that we came up with is detect and protect. In collaboration with a few companies, Hive Biosciences and Biomerica, which is based on Irvine, the idea was to develop detection systems that can be used to protect war fighters in the public.
We really wanted to focus on developing a rapid airport diagnostic because there's not really anything that exists right now. Something that where you can just walk through the terminal and they know whether you're positive or negative within 5-10 minutes. What we want to do is try to combine our rapid nucleic acid diagnostics with point-of-care instrumentation that can be used in airport settings, and in collaboration with Biomerica, get the manufacturing and distribution of this system. This is something that we're working on now, we have a lot of ideas and how to innovate this, and this is in progress right now.
With that said, that's basically my last slide. Just want to thank you for your time, I'm really happy to be here, I really want to give a shout out to the lab and all of the people with the green arrows here that have contributed to the detection system that I talked about today. Specifically Dan Brogan, and [inaudible] and Calvin Lin who led the project. Also our collaborators the Komives Lab and the Knight Lab, specifically Pedro Belda- Ferre, who actually was instrumental in helping us get patient samples to test the system. Our funds, we also got some Seed funds at UCSD through their Emergent COVID-19 related research to help accelerate this project. I think we're going to have a lot more research in this area of diagnostics and I think it's a really important thing to continue developing. Thank you so much.
Thank you Omar. That was a great talk. Next we will hear from chemistry and biochemistry professor Rommie Amaro, who will present captivating insights from her highly publicized work using computational microscopes that provide unprecedented views of the SARS-CoV-2 virus. Rommie scientific interests lie at the intersection of computer aided drug discovery, and biophysical simulation.
She received her BS in chemical engineering, and her PhD in chemistry from the University of Illinois at Urbana-Champaign. She was an NIH post-doctoral fellow with Professor Andrew Mccammon at UC San Diego from 2005-2009, and started an independent lab at UC San Diego in 2009. She is the recipient of an NIH New Innovator Award, the Presidential Early Career Award for scientists and engineers, the ACS COMP OpenEye, Outstanding Junior Faculty Award, the ACS Kavli Foundation Emerging Leader in Chemistry, the Cowan Hans award, and the 2019 Gordon Bell Special Prize for COVID-19. Welcome Rommie.
Hi everyone. My name is Rommie Amaro, and I'm here today to tell you about our work to use computational microscopy to study SARS-CoV-2. Some of you may have already heard about her work in a really beautiful New York Times piece that went to press in early to mid October.
In this article, they presented some of the images that I'll tell you about today, as well as the work of many scientists around the world as we've really raised to try to understand the molecular piece parts of the virus, to really get a better understanding of how it infects humans, and the spread of disease. By now everyone I'm sure is already familiar with the SARS-CoV-2 virus, I'm showing you one of our renditions of that virus here. What I want to draw your attention to is what it looks like. It basically looks like a golf ball with pinpoints sticking out of it.
Those points are basically what we call the spike proteins. We are very concerned with the spike proteins because they sit on the outside of the virus, and so are the first point of contact at the virus has with the human cell. We and others are very interested in understanding, how these proteins work, and how they actually attach to and infect, the human cell.
It's also one of the major antigenic components of the virus. As such, some of you may be familiar with already some of the vaccines which indeed actually encode for this particular protein. During the SARS-CoV-2 infection process, one of the things that needs to happen, in fact, one of the really first events is that this so-called spike protein that I just told you about needs to come into contact with a protein on the human cell. This protein I'm showing here in yellow. This is called ACE2, this protein here that's white and blue is the spike protein. Here in the middle panel you can really see how they come together and make this very close, what I like to call a handshake between the virus and the host cell.
One part of the spike protein that we and other researchers are particularly interested to understand is this light blue bit in the middle. This is called the receptor binding domain or as it's often referred to just by its initials is called the RBD. This is really the main point of the virus that comes into contact with that human cell receptor. So we're going to take a deeper view into this particular part of the protein. In my group, what we are doing is actually using computational methods to explore the fine details all the way down to the atomic level of the virus and the spike protein.
One of the really amazing things about computational methods nowadays is that, we basically can treat them like what I like to consider a computational microscope, where we can pull together datasets from different experimental techniques. So different structural datasets like cryo-electron microscopy and crystallography, together with things like mass spectrometry and genomics in order to build highly detailed, incredibly accurate, computational mimics of biological systems. Then all we're doing is approximating that system down to its many atoms and watching how they move over time. We do this through simulation methods. That basically approximate Newtonian physics. One of the really beautiful things about this, I mean, aside from the math, which can get slightly complicated, but it's really not so bad.
But what this technique allows us to do is to get new views into the virus that we cannot achieve with current experimental techniques. What I want to tell you about are some of our findings as we've used this microscope to learn new things about the virus. But before I do that, I just want to show you what these simulations show us.
On the left here I'm actually showing you again a different view of that really important spike protein, that blue bit, the RBD that I was telling you about. You can see it's still showing up as blue there. I'm sure you're noticing in this movie, these little balls that looked like ornaments hanging off of a Christmas tree and they're wiggling around. One of the places where computing has a lot to give is into understanding something called the glycan shield.
That's what these little ornaments or these little sort of balls hanging off of the protein actually are. The cool thing about this technique is that experiments aren't able to actually see what those sugars look like. These are actually a type of sugar molecule that we have in the body and also on viruses.
But we can't see them with these simulations. So this gives us this unique view. We're really interested to understand what these sugars are doing and how they impact the biology of the virus. One of the major outcomes was our work and one of the reasons why it received a lot of attention was because we showed the world for the first time what the spike protein really looked like. The experimentalist, basically what they see is what it looks like on the left.
This picture where it's just light blue and you can see this is the spike protein at the bottom here is the viral membrane. When we bring in our simulations, on the right side is what it actually looks like. All of the dark blue puff balls that you see, those are those sugar molecules that are invisible to the experiments, but with computing, we can show. Researchers were excited to know obviously what it looked like. As you can see here, the sugar molecules, they decorate or mask almost the entirety of that spike protein.
That's exactly what they were largely believed to do. They act actually as a glycan shield. They call it a glycan shield or a sugary mask that basically hides the viral proteins from the human immune system. So knowing where these sugar molecules are, as well as where they're not, because where they're not located reveal vulnerabilities of the spike protein. This is information that's really critical to, for example, the design of novel drugs or where we want to try to find molecules that will bind into these sites that are exposed. One of the other cool things that our work showed was that it actually showed the world why the spike protein has to undergo a particular conformational change.
Just showing you here it turns out one thing that we learned was that the spike protein actually has two different modes. It has a hidden mode or a stealth mode or a defending mode that I'm showing here on the left. Where those sugar molecules are basically completely covering that part of the protein that needs to make contact with the host cell. But then it has another mode. This is what we call an attacking mode or an exposed mode, where it basically lifts that important part of itself, that infectious bit known as the RBD.
It lifts it up above the glycan shield. Therefore, it actually can go ahead and infect the human cell. The thing that we think is really neat about this, is that this is a finding that if you didn't know where those sugars were located and what they were doing. If you didn't have the information about the sugars that we were able to get from computation. You wouldn't have any idea that it's actually hiding in one mode and revealed in another.
The other thing that we found that was pretty surprising was that we showed that there were two glycans, in particular, two sugar molecules I'm showing you here. They're shown in sort of swollen ball structures. That these two sugars actually hold up a top that spike up in the open conformation. It's not only that those sugar molecules are basically hiding or masking the virus from the human immune system. But they also act as part of the viral weaponry itself by locking and loading the spike for infection to the host cell.
This was something that not only as if we were the first to show for SARS-CoV-2, but to our knowledge it's actually the first time it's been shown for any viral protein. So it's quite exciting this new love for glycans that are simulations together with experiment established. That's just the beginning of what we're doing.
We've also significantly expanded the scope of our simulations to actually study the spike protein in the entirety of the viral envelope. What I'm showing you here are some of the largest simulations that have ever been carried out for biological system. They have roughly 300 million atoms and are running on the biggest supercomputers in the world.
But this is like opening entirely new dimensions to the study of virology, I suppose study of antibodies, and how these viruses actually infect human cells. Then I'm sure that many of you have also heard a considerable amount about different mutant forms of the virus mutation variance of concern. These type of computational methods that I just told you about can also be used to very rapidly assess the potential impact that these mutations could have, not only for transmissibility, but also for the ability of antibodies and vaccines to be effective.
With that, I just want to thank a highly integrated global team who's carried out with me and my group a tremendous amount of research over the past 10 or 11 months. This includes the group of Elisa Fada, shown here at the top. A terrific glyco-chemist, and glyco-biologist at Maynooth University, in Dublin.
Members of my own group, particularly Lorenzo Casalino, Zied Gaieb, and Abigail Dommer, who have just worked tirelessly over the past number of months, and all the folks I've shown here, including our experimental collaborator, Jason McClellan, he's one of the co-inventors of the Madonna vaccine and has been really key in helping us to experimentally validate a lot of the things that were coming out of experiment, of the simulations. Thanks for your attention. Thank you Rami for that great presentation. Next we'll hear from Biological Sciences along, Dan Kolk of 1983, who was a senior diagnostic advisor with Tunnell Consulting Group Incorporated, supporting borrowed diagnostics, who will address the future of at-home diagnostic testing in response to COVID-19. Dan has over 20 years of experience in the in-vitro diagnostics industry and received his BA in Biochemistry and Cell Biology from UC San Diego in 1983, and his PhD from Arizona State University in 1992. His PhD thesis focused on gene regulation of immune responses by cytokines. He did his post-doctoral fellowship at UC San Diego lab of William Wachsman. Welcome Dan.
Thanks. My name's Dan Kolk. I'm going to thank the division of Biological Sciences for inviting me to speak about my area of expertise which is in diagnostics. I've spent my entire 25-year career in the molecular diagnostics industry. I'm going to talk to you about some of my beliefs and thesis about how I think COVID is going to change the diagnostic testing paradigm. I want to just give it a disclaimer before this talk.
What I think is going to happen is that, you've seen it already, in fact, the pandemic and the medical diagnostic industry's response to it has shown some real issues with how we do medical diagnostic testing, particularly for infectious diseases. This is forcing us to rethink the model of how people access infectious disease testing, and so we're going to see a shift and it's on its way. It was already on its way before the pandemic, but the pandemic's going to speed it up and we're going to see a shift at-home testing. This shift, at first it's not going to be obvious, but this is really a cusp of something much bigger where the medical consumer who already wanted to be more involved in their medical decision-making and control of what's going on in their body.
They're going to be empowered with far more information than was thought possible just 30 years ago due to technological advances and other factors which I'll go into. The goal is to make at-home diagnostics testing as common as a toothbrush. But everyone knows that's a high bar because tooth brushes are pretty inexpensive and they're pretty easy to operate. You can't say the same about diagnostics. But let's get to what's going on right now in the United States in COVID diagnostics.
This is a graph from early March 2020, showing the test per 1,000 people, both nucleic acid tests. I'm going to refer to as nucleic acid amplification tests. Those are what people commonly refer to as PCR, but PCR is not actually the right name because there's lots of ways to do nucleic acid amplification tests. I'm going to call them NAT-test. This graph also includes antigen tests. NAT tests are extremely sensitive.
It's a state of the art. But as you were told in an earlier talk, they're complicated. Up until recently they were very complicated.
Antigen tests are far simpler and far less expensive, but they're not as sensitive. Antigen tests are easy to access, easy to manufacture, but not quite as sensitive as nucleic acid tests. So what happened? We all know that there wasn't enough capacity for testing and there still isn't. We really needed three to five times as much SARS-CoV-2 testing as was available. The virality is the surge of testing was so rapid and so large. There simply wasn't infrastructure in place both at the laboratories at the manufacturing level.
It was just not contemplated that this kind of demand could occur so rapidly. By the way, the vast majority of infectious disease testing is done in large reference laboratories in large hospitals and medium-sized hospitals. The tasks are provided by large and medium-sized manufacturers. Even if those tests were available, the laboratories couldn't process samples fast enough because they didn't have the experienced labor, they didn't have the testing infrastructure, and they didn't actually have the devices used to collect the samples. There's one other thing that was key in this, is that most people don't know the United States, the most of the world medical diagnostics, referred to as IVD or in-vitro diagnostics, they have to go through a regulatory cycle.
In the United States, it's controlled by the FDA, they have to go through something called design control. That requires a very stringent process of designing assays, testing them, validating them, and then scaling them up for manufacturing. That just takes time. You can't flip a light switch and have that happen overnight. Here's two items which caused pitch points early in pandemic.
On the left is how a nasopharyngeal swab is collected. You can see this is a medical personnel sticking a swab into the back of the sinuses and this at the time was considered the gold standard and still is considered a gold standard sample. I'm going to show you some data in a second from saliva. We're now getting good data that suggests that saliva probably is as good as nasopharyngeal swabs for running these nucleic acid or NAT tests. There was a swaps shortage.
The manufacturers of swaps were not prepared for the surge. They have responded and they are now making far more swabs. But that right there created backlogs, and secondly, as you can see you need a trained medical personnel person to take the sample, and thus, you created another pinch point where people had to go into a laboratory or into a physician's office to get the sample taken and there just was too many people trying to do this too quickly. On the right are some of the reagents used in the CDC assays. The early assays were required reagents called RNA extraction reagents.
It's a group of pretty specialized chemicals to extract the nucleic acids out of the samples and there were shortages of that, and that required skating of the manufacturers and that took time. Here's some data from a recent New England Journal of Medicine paper as. In December of 2020 where they compare the viral load in saliva samples, these are matched samples to the viral load of SARS-CoV-2 in nasopharyngeal swabs, and what the paper concludes, is basically, there's more viral RNA in saliva. Think about it, saliva is a very easy sample to collect.
People can actually self-collect, you just spit in a tube, and that sample could be either mailed to a site, as you're going to see later, can actually be tested by the person right there. They don't need specialized equipment to collect a sample, and they don't need medical personnel to collect the sample. These are the platforms that nucleic acid tests were run on.
On the left are what we call the medium-throughput, or sometimes, depending on the configuration, people can run these systems fairly high volume. But the upper left instrument, that's a nucleic acid extraction instrument. That was where the reagents required for that were in short supply early in the pandemic.
On the right of that is a real-time PCR, Thermal Cycler. This is the equipment that many public health labs in this country use, where they have a separate instrument to isolate nucleic acids. Then they mainly pipette that into what we call 96-well plates and run it on the instrument on the right. Well, when the volume surged, you need something called a robotic pipettor or liquid pipettor, that's shown at the bottom. There's shortages of those.
The infrastructure for these labs didn't have the equipment they needed, they didn't have the reagents they needed. The problem was this concentrated, everyone brings their sample to two local sites for testing, got overwhelmed. Now on the right are instruments that are high-throughput instruments.
These instruments have been around for a while, but they're not as widely dispersed, they're relatively more expensive than the instruments on the left, but they're out there. They're just not as widely in use as we needed, and if more of these had been available and the reagents had been, a test had been ready, we could have made far earlier major impact in the pandemic. The US government is supporting these manufacturing scanning efforts. BARDA, NIH, Department of Defense are all involved in helping scale manufacturing.
The current system we need it, there's no going back from where we are. We have to have centralized high volume and medium volume testing variety of other types of testing, not just SARS-CoV-2, but a decentralized testing model could help alleviate the bottlenecks. If people could do the testing at home, they wouldn't have to get into the physician's office or testing laboratories, and the facts are, that people have been doing at-home testing for long times anyways. There's been, home pregnancy tests are actually basically a diagnostic test, blood glucose monitoring, that's basically a diagnosis tests. These are things that have been around for a long time. These forces I've been referring to, is that, the miniaturization of molecular diagnostics technology, the rise of telemedicine in this pandemic, they all are now forcing people and allowing or enabling people to do testing at-home, when the tests are available, they now are becoming available, and I'll talk about that in a subsequent slide.
But the fact of the matter is, people for a long time have been interested in the testing they're being done on their body, they want to be involved in decision-making, and all these forces are driving diagnostic testing to the home, and in the long run, this will be a good thing for the next pandemic, and there will be a next pandemic. There's been three, SARS species or sub-species have arisen in the last 20 years. There's no reason to think this is the last one we're going to see.
But for other types of infectious disease testing like sexually transmitted diseases, hopefully, someday, those tests can be done at home too. Recently, the FDA has approved, issued EUAs, so Emergency Use Authorization for two self-administered self-collected at-home, SARS-CoV-2 test, one's an antigen test, and the second one is a nucleic acid test. Now this is the first time that we've actually had a nucleic acid test at home, approved. You have to get a prescription for it, but you collect the sample, you run the state of the art Nucleic Acid Amplification at home for an infectious disease. Now, this obviously still got some constraints, there's manufacturing constraints, but it's a great first step to get to decentralize the testing.
The second test that I referred to that received the EUA, is an antigen test. These tests are much simpler, and that side of the diagnostic industry, they are capable of scaling very quickly. They have large volume, but these two kinds of tests together are really going to make a dent in the shortfall in nucleic acid testing.
But then there's this other area that could be of very high importance in regards to the vaccine and these variants of concern. That is in the idea of immunization passport. There's been a lot of talk about this, and let me just say from the get-go, the science is still out on this, it's not cleared, this is going to be real, it's not clear that just having antibodies to SARS-CoV-2 means that you're not infectious, or that you may not get infected by another variant, but neutralizing antibody assays this time, looked to be the best hope for immunity passports. These types of assays, I think someday, we may see these kinds of antibody testing. The neutralizing basically means, these are antibodies that they now block the virus from infecting cells. At this point, we believe that the best indication of immunity, but there's a lot of science still ahead.
That's all I have. Thank you. I really appreciate the opportunity to present this. I'm going to close today's session. I want to first thank each of the panelists for their really inspiring talks.
This, showing how innovation can happen both on campus, and in leadership, and in science, and in the regulatory agencies and getting more tests approved. Thank you all, it's been really inspiring to hear from you, and thanks for all of our attendees for joining us this afternoon. [MUSIC]
2021-03-30 11:42