Quantum science: from philosophy to technology
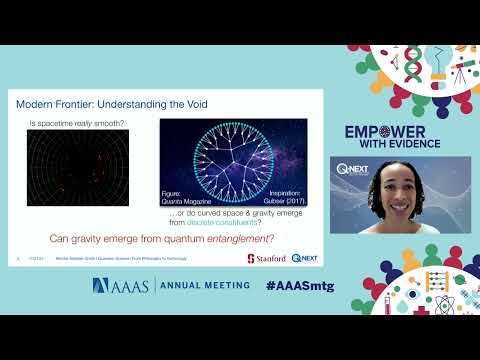
(bright music) - Hello, I'm Sylvia Zorzetti, a senior engineer at Fermilab, and ecosystem lead for the Superconducting Quantum Materials and Systems Center, a Department of Energy National Quantum Information Science Research Center. I am the moderator of the full session, scientific session Quantum for the People, connecting quantum information science and society. Thank you for joining us. This presentation is one of the three prerecorded videos focusing on the speaker's material before all the panelists come together in February during the live discussion. In this video, our panelist, Monika Schleier-Smith, will discuss quantum simulation and the shape of spacetime. Later in this video, Monica and I will delve into how a future quantum workforce can extend this research field.
Monika Schleier-Smith is an associate professor of physics at Stanford University, a MacArthur Fellow, and a member of Q-NEXT, a Department of Energy National Quantum Information Science Research Center led by Argonne National Laboratory. Her next mission is to develop the science and technology for controlling and distributing quantum information, and maybe pivotal discoveries in the U.S. competitiveness in quantum science and engineering.
Welcome, Monika. - Thank you, Sylvia. So the title of my talk today is Quantum Science: From Philosophy to Technology.
Now, I'm an atomic physicist, and when I think about quantum technology, the favorite picture that comes to my mind is something like this. So here are actually some pictures from a few different labs around the world showing individual atoms that are optically trapped, each one at the focus of a laser beam. And it's possible with modern day technology to really have control of these atoms at the single particle level, and the ability to precisely position them and manipulate them. And this is really a powerful technique for a number of different applications ranging from extraordinarily precise measurements of time. The world's best clocks are made of these trapped atoms.
Another direction that's being pursued is using these atoms as the individual quantum bits of future quantum computers. And we're still a ways away from the point where... We have a universal quantum computer that is a multipurpose device like the classical computer on your desktop.
But what's somewhat closer to fruition than an area of active investigation is an area known as quantum simulation, which asks, "Can we use well-controlled systems like these, precisely controlled atoms in the laboratory, to solve a certain class of problems that are difficult for classical computers?" And in particular, those are often problems that are inherently quantum mechanical, like problems surrounding the properties of materials governed by the quantum mechanics of interacting electrons. If one can build a well-controlled interacting quantum system, one can use it to explore from the bottom up how one might engineer the properties of materials and learn things that one cannot calculate on classical computers. So that's the area of simulation. When I look at sort of these rich possibilities enabled by this precise control and detection of single atoms, I think it's kind of amazing how far we've come from an earlier stage in human history where the concept of an atom itself was actually controversial. So if we, in fact, kind of look back to ancient philosophy, we owe it to the Greek philosopher Democritus the entire concept of the atom as the foundation of matter.
So at the time, there was this revolutionary idea, eloquently phrased by Democritus, that things like sweetness and bitterness, hot and cold, color, these are all by convention as he says. "In truth, there are but atoms and the void." So this idea that atoms are the fundamental building blocks of these rich emergent properties like taste and color and temperature, that's an idea that is obviously very powerful for our understanding of the world today.
And putting it into in sort of modern language, there's this concept that macroscopic behavior is something that emerges from the microscopic configuration of individual atoms. So today, we understand that very well. We know how to harness that idea for technology. But one other concept that appears in this quote from Democritus besides atoms is the void, and actually the void is something that even today there is much mystery and philosophy about understanding the void. So what do I mean by that? So Einstein's theory of gravity describes spacetime.
We know that space can be curved by matter. And we have this picture that spacetime is something that is smooth, whereas we said matter is something that's composed of these discrete constituents which are atoms. And one question of really modern inquiry in physics, and particularly in theoretical physics, is is spacetime really smooth, or is there here also some underlying microscopic description in terms of individual constituents that we just can't see yet with current technology. So how might spacetime and curved space emerge from microscopic constituents? Well, here's kind of a picture, due to the artist Escher, that conveys this idea. So this is a depiction of a hyperbolic space, and the way that you should think about this is that all of the fish in this picture are actually the same size, but they don't all look the same size. Some of them out at the edge look very, very tiny, but that's just because we are projecting this curved space onto my slide.
And so really, this is saying the circumference here is exponentially larger than the diameter. We're in a space with negative curvature. And so here, the microscopic constituents are fish. No serious physicists thinks that our space timing, our universe is composed of fish. But the type of picture that we might have in mind as physicists is maybe there are some real microscopic constituents are, let's say quantum bits.
As an atomic physicist, the picture I have in mind are individual atoms. But some sort of bit of information, here they're shown as these blue dots around this circle. So that's sort of in one possible model, really the microscopic building blocks. And the concept is that perhaps curved space and gravity emerge from these microscopic building blocks, sort of like a flow of liquid emerges from individual atoms. But here, they emerge as a consequence of a phenomenon known as quantum entanglement.
And roughly speaking, this idea of entanglement means that these individual quantum bits around the boundary here, at some point in their history, they interacted in some way they became correlated. And in the future, there are some correlations in their behavior, which are described by these lines. These lines say that if I measured some property of an atom here, it might tell me something about another property there. There's this fabric of entanglement. And it's curved because again, the circumference of this tree is exponentially larger than the radius of the circle here. Okay, so can gravity and curved space emerge somehow from this phenomenon of entanglement? That's a very deep question.
And there's really elegant theoretical work done to explore this, but tackling this problem in experiments is actually extremely challenging. If you want to start to explore this question in experiments, there are kind of two approaches you might take. One is doing better and better precision measurements of our universe and trying to access regimes where both quantum mechanics and gravity are playing a role, placing massive objects in so-called quantum superposition states where one fundamentally doesn't know is the object here or there.
Doing exquisitely precise measurements of time or accelerations in regimes where gravity is playing a role and can affect the rate at which a clock ticks. So that's one approach. A complimentary approach is to say, rather than trying to directly see evidence of per some possible quantum phenomena and gravity in our universe, can we build model systems in the laboratory starting from the microscopic constituents that are individual quantum bits, and asking if we let them interact and build up correlations, are there situations where that phenomenon of entanglement arises and looks like curved space and gravity? Either approach that you want to take, and I'll focus on the second one, but either approach that you want to take requires very sophisticated quantum engineering. So the ability to build quantum systems in the laboratory where we have a high degree of control among the interactions and the resulting correlations between the constituents. So the quantum system, one of the quantum systems that we work with in my laboratory is one where those quantum constituents are atoms. And in order to control their interactions and correlations, we actually let them interact with light.
And we like light because photons are a convenient way of conveying information between distant atoms. And in particular, we use photons to sort of let any atom in our experiment talk to any other atom. And the concept is, I showed you before we have extraordinary control over, for example, patterns of light that can be used to trap atoms and various geometries.
Here, we'll be interested in using our control of laser light to also control the interactions between the atoms. The experimental setup where we do that looks something like this. So conceptually, we essentially have two mirrors facing each other, a few centimeters apart, and in the lab that looks something like this. And here, what you're seeing is a cloud of atoms that are actually being cooled by laser light, to a temperature 10 million times colder than room temperature. And we optically trap them between those two mirrors, and what we have in the end is an array of about 20 little clouds of atoms.
So this is not an individual atom. Each of these spots is a few thousand atoms, and we're interested in controlling the way that they talk to each other with the aid of light. And so to give you a flavor of how that might work, so first of all, we can encode some information in each individual atom. In our atoms, we do that by letting using sort of three internal states, I'll call them the -1, the 0, and the 1 state.
And so each atom has these three possible magnetic states. And if we want two atoms to talk to each other, we can send in a laser beam that allows light to bounce off of one atom, and then off of another, and change the states of these atoms in a correlated fashion. So for example, in this case, we turned two 0 atoms into a -1 atom and a +1 atom. But as long as there's no information that leaves the system about whether it was the left atom or the right atom that was in the plus or the minus state, generically, this process could give rise to the phenomenon that I mentioned earlier known as entanglement, where one has some so-called quantum superposition of the left atom being in minus and the right atom being in plus, or vice versa. That is to say, if one can make the state, it's sort of fundamentally unknown and unknowable which atom is in which state, but if you were to measure one atom, the other one's state would be precisely determined.
So that's this concept of quantum correlation or entanglement. This general approach of using light to generate entanglement among atoms is something that's quite well-established. And in the simplest case, what one has, if one places these atoms between the mirrors and lets photons bounce around between them and generate correlations, every atom can talk to every other one.
And that generates a form of entangled state that actually is very useful in a context I mentioned before, which is precise measurements of time. And in fact, one can generate a form of quantum correlation that even improves measurements of time beyond what's possible in the absence of those correlations. But what we are actually particularly interested in is going to richer structures of correlation, and so a focus of our recent work has been on programming the connectivity of which atoms talk to which others using the high degree of control that we have over the laser light. And so just to give kind of a flavor of what we can do, here's a little gallery of a few different sort of graphs of connectivity that we have made simply by controlling the modulation and time of the intensity of light that we send onto the system.
And so depending how we modulate our laser, we can make the atoms, even though they're sitting physically in a little chain, we can make them talk to each other as though they were connected in a ring, as though they were connected in some sort of cylindrical geometry, even as though they were connected along a Mobius strip. So these are all things we can do simply by the high degree of control we have over the light field that lets the atoms talk to each other. So now, we would like to ask whether there are situations where some notion of maybe curved space or gravity could emerge from the correlations among the atoms. So to explore that, we built sort of a model system where we compare two different scenarios. So the green dots here are our little clouds of atoms, and the blue bonds represent the way that we program them to interact.
In the situation on the left, the strongest interactions are essentially between the neighboring clouds and we connect them essentially in a ring. And in the system on the right, we do something rather different where the strongest interactions are actually between the clouds that are furthest apart, and there are some weaker interactions at other length scales. And so we turn on the light that generates these interactions, we let the atoms talk to each other, and after some time we ask, "What do the correlations look like?" And to explore that, the first thing we do is we basically, so we analyze the data, we make a plot where we draw a little dot here. The numbered dot represents a particular cloud of atoms.
We draw them so that if they're strongly correlated, we draw them close to each other. And then, furthermore, we can actually kind of emphasize that by drawing a bond between the clouds that have the strongest correlation after they've interacted. And here, those are the nearest neighbors and we form this ring.
But we can also go one step further and say, "Okay, so these are correlated in pairs. And if we repeat that process, draw bonds where the correlations are strongest, until everything is connected, what emerges is precisely this tree that was a model for a hyperbolic space with negative curvature." And so here's really an example of starting from the microscopic degrees of freedom that are these little atom clouds allowing them to interact and build up correlations and coming up with a picture where that system is best described by some emergent geometry featuring curved space, a discretized version of curved space with some microscopic underpinning and quantum mechanics. So that's a toy model. I can't say that this is how our curve space arises in our universe, but the fact that we can even begin to explore that possibility in the laboratory really builds on the high degree of control that we have with modern day quantum science experiments. And that high degree of control is being exploited.
I should mention, I talked about connections to fundamental physics, but it's also being harnessed for a range of applications with promise for future technologies ranging from better clocks, sensors, computers that harness the phenomenon of quantum entanglement to actually harnessing the high degree of control of interactions to directly program in specific computational problems that could have technological applications. So there are lots of exciting possibilities in this realm and also plenty of challenges remaining to be solved. And so to solve those challenges, one of the things that I am very passionate about is also equipping the next generation with the knowledge and the perspective to really get into these cutting edge problems in quantum science and engineering. And so in the classroom over the past few years, I've developed a course where we're introducing college freshmen without relying on prior background in physics to really cutting edge concepts in quantum information and quantum technology.
So with that, I want to actually thank AAAS again for hosting this scientific session on engaging more people in quantum science and engineering and hand it back to Sylvia, our moderator. - Thank you, Monika. That was a very interesting presentation. I have a few questions for you. So my first question is you have described how, what we will call basic science.
It brings together quantum physics and spacetime. How does this lay the groundwork for applications and what kind of technology could be advanced through this? - Yeah, thanks for the question. I touched on a few different directions in the last slide, but I didn't have a chance to say much about them, so let me go into a little more depth on a few of those. So fundamentally, the key sort of ingredient that I showed you for being able to do these simulations connected to fundamental physics is the ability to control interactions between the constituents of a quantum system. And one direction where that is equally relevant and interesting is in the realm of computation.
And in particular, there's a range of computational problems, sort of optimization problems that are encountered in the real-world, things like route scheduling problems, or traffic routing, routing deliveries, that actually can be mapped to. So it's some optimization, you're trying to minimize some cost function. And they can be mapped to the problem of minimizing the energy of an interacting physical system. But to take a particular real-world problem, I'm trying to optimize this particular scheduling task and to sort of map it to the hardware, what you need is to build sort of the right structure of interaction so that minimizing the energy of your physical system corresponds to minimizing the cost function of that classical problem. And generically, that requires sort of exotic interactions that might not just be between neighboring atoms in some chain.
And so this ability to have this high degree of control over interaction graphs is something that could be applied in that area of optimization. And that is interesting because it's sort of a step earlier than sort of the larger goal of building a universal quantum computer that will solve any problem. It's can you solve this certain class of problems that naturally map to energy minimization? But also then for the goal of universal computation and for broadly in quantum technologies, the fundamental enabling resource is the resource of entanglement. And I touched on that, but I wanna maybe say a little bit more about that.
So entanglement essentially means that the state of the quantum system, some collection of quantum bits, it's not fully described by knowing for each individual bit. Is it in, let's say, the 0 state or the 1 state. But one really needs to keep track of correlations between the states of all of these different quantum bits, and that means that fitting the description of even a small quantum system of say 50 to 100 quantum bits onto a classical computer, this rapidly becomes impossible to fit even onto the world's largest super computer. So that gives us a flavor for sort of why quantum systems, on the one hand, the problems that are inherently quantum mechanical are hard to solve on classical computers, but why also we think that if we have a high enough degree of control over these systems, we can solve potentially problems that classical computers can't. But that all comes down to being able to generate those correlations, to generate this resource of entanglement. And to understand that resource, and that's where having some simpler picture in terms of are there cases where we can understand it, in terms of pictures like spacetime, curvature and gravity, maybe that helps us also understand how to model and describe these quantum systems that are inherently difficult to compute the properties of.
So I touched on computation. And I'll just, if you allow me also mention, at least one other sort of key direction, which is quantum sensing and quantum precision measurements. I emphasized that engineering interactions and correlations can be a resource for improving precision measurements. And that's particularly when one gets so good that quantum uncertainty is the limit to one's measurement of time or acceleration or a magnetic field. And there are applications then in areas from navigation. GPS relies on atomic clocks.
More generally, the ability to measure accelerations is valuable in navigation. The ability to measure magnetic fields is important for everything from materials, characterization, to biomedical applications. And when everyone is trying to do that with the highest possible precision in the fastest possible time with the highest possible spatial resolution, one starts to try to really exhaust all of the resources at one's disposal, and the phenomenon of entanglement can be a resource that can help there.
- Thank you. In your presentation, you also mentioned efforts toward workforce development, so this question is for undergraduate students that are listening to this presentation and to this talk. So what if they want to get involved either in basic research or in applied science, how will you advise them? There are classes that they should take, or what kind of measures should they take? - Yeah, so great question. I think one of the exciting things about the stage we're at in the field is that we need expertise from so many different areas. And I sort of touched on the fact that there are connections to certainly computation, but applications in chemistry, biology, material science. And so I would advise students to think about gaining the fundamental background in quantum mechanics, which requires some physics background, but also potentially something else, be it, computer science, engineering, chemistry, and bringing those things together so that, one, you have sort of the foundation and quantum mechanics but also the exposure to what are the problems that we want to use quantum technologies to solve.
And so I think it's a really great opportunity for students with a range of interests to get that sort of... I would advise getting a mix of experience, learn quantum mechanics, take physics, but also learn something else so that you can contribute something really unique to the field. - Thank you. So one slide in your presentation, you mentioned Escher, the art of Escher, which is a nice hook for drawing people into quantum science also in a visual way.
Are there other innovative ways that we can use to explain quantum science to people and engineering to the people? - Yeah. So on the one hand, I always love visuals to help me imagine quantum entanglement, quantum uncertainty. On the other hand, it also is really useful to get also the sort of rigorous foundation to be able to understand how a quantum algorithm would work. And for that, one of the things that I've found really satisfying is realizing that although it typically takes two or three years of undergraduate physics to get to the point of a full course in quantum mechanics, one can really understand qubits and quantum measurement and quantum circuits using, actually essentially high school level mathematics. And so it's been really satisfying for me to develop a course where through a combination of minimal background, high school level background, and also some hands-on activities using optics to understand the phenomenon of quantum measurement, we can really get to sort of rigorous ideas in how quantum mechanics is used in algorithms and fundamentally secure communication of information that's eavesdropper proof because of the laws of quantum mechanics.
These are actually all things that can be taught without years and years of physics with just a basic mathematical foundation. - And now, still talking about innovations, but also bringing workforce development in, so what kind of innovations we as a quantum information science research center can bring for expanding the quantum workforce? - Yeah, so I think one thing that we can bring is potentially bringing together different communities that have things to learn and things to contribute to each other. So that can be academia, national labs, and also industry. And so as in academia, we're thinking about innovative ways of teaching quantum information.
In industry, there may be people who are coming from a slightly different background and want to enhance what they can do with quantum science and technology or contribute to that endeavor. And there is a possibility also for students from academia to go to industry and experience actually what are the challenges in scaling quantum technologies really see that industrial perspective. And so I think this is one thing we can do is bringing together these different participants in next generation quantum science and engineering. - Last question. What book on quantum science and spacetime will you recommend to people to read? - Okay, so I found it hard to choose just one, so I'm gonna list four, but I'll be quick about it.
So when I teach, if you're sort of, because different people have different styles, so if you want kind of a textbook, the textbook I use for college freshmen is a wonderful book by Eleanor Rieffel called "Quantum Computing: A Gentle Introduction." If you want more sort of, again, something conversational, there's a great book by Scott Aaronson called "Quantum Computing Since Democritus." And I have to mention that 'cause I had that picture of Democritus on my slide. And that gives a wonderful broad perspective on the field of quantum computing, as well as the philosophical aspects. And lastly, my colleague Lenny Susskind has a series of books that convey topics ranging from quantum mechanics to gravity, to the interest of general reader. So he has a book called "Quantum Mechanics: The Theoretical Minimum," and he has a book called "The Black Hole War" that I myself have used to gain some perspective on what led to these questions about whether gravity fundamentally might emerge from quantum mechanics.
- Thank you again, Monika, for your presentation. - Thanks, it was great to be here to talk about quantum mechanics, gravity, and how this work could drive quantum technology. Thank you to the audience and to AAAS for hosting this talk.
- Thank you again. A reminder that this is only one of the three spotlight videos focusing on video work of the speakers before they all come together in February in the live panel Quantum for People connecting quantum information, science and society. Please check the AAAS annual meeting website for the panel's date and time. Again, thank you, Monika, and the audience. We will see you live in February.
(bright music)
2022-07-12 15:29