Jeremy O'Brien: "Quantum Technologies"
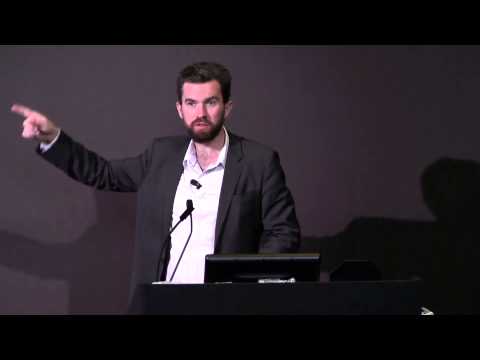
Jeremy. Is the. Director. Of the center for quantum, photonics in, Bristol, which, is sort of ream it's, pretty fair to say, one. Of the if not the world's. Most important. Place for doing. Photonic, implementations. For quantum, computing, Jeremy. Originally, is from Australia. And, we got his PhD and, also. Spent many. Years as, a senior. Researcher. On cost. Related, topics. And confined, electrons. And organic, structures. Superconducting. Devices. And of course photonics, and. Folks. May know that the UK, just announced. I think it's half a billion pound, the, UK pound. Dollars. Okay. So half a million. Half. A billion, dollars. On. A new, quantum, computing initiative. And. Some. Of those, funds are probably going to the University of Bristol to first as a work you, guys are going to hear about and, that of course allow. Us to scale up initial. Prototypes. In interesting. Ways and that will briefs, I think additional, life, into. The overall field and also will, create. Broader. Interest, around, quantum. Photonic, devices, so, Jeremy very interested, to hear what you are going to do right thanks very much happen. So. Firstly thanks very much for the opportunity, to, to, be here. And. Tell you a little bit about what. We're doing in Bristol. In in, photonic. Quantum technologies. Generally. I'm going to focus on. On, quantum, computing because I think that's probably what you're most interested in, but I'll touch on these other areas on the, way through. Before. I get going I need to acknowledge, that there's quite, a large number of people at Bristol and beyond that, have. Done the work that I'm going to describe and I'll try to highlight who they are as. I go, along. So. Before, I get. Going I just want to put up a quick advertisement, for this Center for doctoral, training in, quantum engineering which. Which. Google apart of whether you like it or not thanks, very much to Hartman, for giving. Us quite a bit of advice and supporting. This enterprise. The, ambition, here is to Train of order. Fifty to a hundred. Graduate. Students, in quantum, engineering over the coming eight, or nine years and the, focus is very much on the engineering so, I think it's all in the title it's about training, a new, generation of of engineers, who understand, the quantum physics but who are, very much focused on delivering, the, technology.
So I. Guess the the message for you is if you want to hope you know if you want to host some. Very, smart young people with. This sort of mindset who will have some some, pretty substantial initial. Training then please sir please get in touch and I think my. Colleague Mark Thompson who's the director of this Center will probably, be sending heart that I am a, request. For projects pretty soon so just bear that in mind okay. So. In. In the usual way. At, Bristol, where we're interested in these quantum technologies, in communication, secure and other types, of functionality that you get by harnessing quantum mechanics in, sensors. Which which. Reached the ultimate precision, limits. Dictated. By the laws of quantum, physics, we're, interested in simulations, in particular. You. Know analog simulations, that are distinct, from a full-scale, quantum. Computer as well as the science that underpins that. Area, and what. I'm going to mostly talk about is quantum computation, I'll mention some of these these highlights that I've shown here where we've we've. Made. A demonstration, of this sort of handheld. Device to bank ATM, machine for example of secure key, growing. We've. Measured the concentration, of a blood protein, using, entangled, photons in a device like this which combines those integral, photons with a microfluidic. Device, and, we've. Done a simulation of a helium, hydride, molecule. Again. Using one of these integrated, devices and as I say I'll touch on those three things a little bit as I go along but mostly I want to tell you about. Our efforts towards, a. Full-scale, photonic. Quantum computer. This, is really my advertisement. For a photonic approach, to these things so I think it's it's fairly clear that there's no there's, no really, feasible alternative than, photons for communicating, quantum. Information over, any distance. Optical. Interferometers, like this that operate in, the classical, regime are. Arguably, the most powerful precision, measurement tools we. Already have and so enhancing. Their precision using, photonics. Is a very natural way and I, think it's fair to say that it's in historical, fact that, photonics. Is has led the way in exploring, the foundations, of quantum physics and then more recently in. Exploring. The fundamentals, of quantum information science through, violation. Of Melonie qualities through entanglement, of multiple systems. And teleportation. And so forth and so, the. Argument would be that if you're doing analog, type. Simulations. That photonics. Is a very appealing way, to do that where you you know you have much smaller scale systems. And, what I'm going to make an argument for is is for photonics, for, you, know a full-scale programmable. Digital quantum, computer.
And. So, I'll. Start just with a with a reasonably brief, background, on, photonic. Quantum computing, and then, tell you about our particular approach, to doing that and try to highlight some. Of the check you know that there's some of the current challenges and the future, challenges to. All of this. So. Just. Briefly. This. Is say this is an article that I wrote with several. Colleagues a few, years back on physical. Approaches, so the platforms, for, quantum computing and of, course we couldn't get away with, publishing, this paper without the editors insisting, that we produced a league table of all of the different approaches. And we were pretty reluctant to do that and we were we. Were made to do that so, you know compare it trying to compare all these different approaches, and I. Guess. The reason that we were reluctant is that this, table is not really the not, necessarily the meaningful, thing, to do and the, reason, that there is this sort of tie is because building a quantum computer is, you. Know has a sort of list of requirements, that are all that, are pretty much contradictory, to one another namely. You need these physical systems that, have very very low noise which means that they're very. Beautifully, isolated, from, their environment and yet, they interact, extremely, strongly with one another if you want to if you want to implement logic. Gates for example they, interact very strongly with your you, know your preparation, and readout apparatus, so there's this sort of contradictory, requirements and. What you find is that invariably. Things go work you know things things look good on several of those requirements and not so good on on others so that. Nevertheless. You. Know even back then it was pretty clear to us that actually, what was what, was important, you know was the ability to operate these things in a fault-tolerant way and and, and actually you. Know realizing architectures. For these, some. For. Any of these approaches, and that that would be a sort, of more meaningful comparison. And, I guess what I'd argue now, is that we're, moving even even, further ahead and I think the arguments, now in my mind are about manufacturability. So, can we really make large-scale. Devices. And this is a cartoon of a, of a kind of a. Large-scale photonic, quantum computer, where, unless you're, a you know photonic specialist, and most of it will be fairly meaningless but the point that I'd like to make at this stage is simply that all of the elements there are. Ones, that are familiar to photonics, engineers they've been used in, telecommunications. And, other applications, for years or decades, that. Those, same photonics, engineers would be a bit daunted at the scale of the whole thing but certainly the components, that are going into it are familiar, to them and what. What you don't see in there is sort of you, know any. You. Know physics breakthroughs, required you don't see any sort of exotic, system some so you know atomic scale, fabrication. Or milli Kelvin temperatures, or ultra high vacuum or, anything like that so it's a it's, a it's, a fairly friendly environment, and the, actual fabrication of these components, is is, done using the same techniques that are used in in. Fabricating, micro electronics, so that's the promise of scalability, in, terms of being able to manufacture their components, and then I think a big challenge.
In Terms of assembly. And manufacture. And. I'll come, back to sort of give. You some details on that now no just this sort of introduction. If you like to the, photonic approach to to quantum computing, I am I I. Was. That I am a iro. Kickoff, meeting, at. In. Maryland, last, week and I uh I introduced. The introduction, by uh telling. Them all that they might have forgotten what photonic quantum computing, is since, I ARP I keep, this out of their programs several years ago, which. Made them chuckle but I I hope, that they. Can also see the the promise of this now and obviously they wouldn't be funding as if if they didn't okay. So the, you, know encoding in the polarization like this is some it's, very appealing my my colleague at Bristol John rarity likes to say that the lifetime of these qubits is at least the age of the universe because the microwave background radiation, is, polarized, now. I guess that's a fair statement you'd need a fairly big working space to take full advantage of that but, intrinsically, low noises is the point here so, effectively. A zero temperature, system, intrinsically, which is very exciting, we, know a lot about the polarization of light and everything we know about the polarization, of light for example is directly transferable, to the polarization of single photons and in fact we can manipulate the polarization, of. Photons, in a similar way it's. Often said that you know we use the same off-the-shelf, components to do this and and, that's the advantage we do use the same off-the-shelf, components but I would argue that you manipulate, a spin with the same off-the-shelf components the difference here is, that you have a very very, powerful. Means, to calibrate these, things very, precisely and, that is simply to send a bright laser beam through, your through. Your device with, exactly the same properties except, its intensity, and then you can calibrate, your. Your quantum systems your, photonic systems very precisely, there's. Plenty of other degrees of freedom that you could use so here you, can see how you go from a polarization. Encoded. Qubit via. A polarizing, beam splitter to a path in coded qubit where you now have a superposition, of a photon in this path or, that path, and. In fact it's this path encoding, that I'm, going to talk about, pretty well exclusively, from here on in. And. Of. Course this there's, always a conservation.
Of Trouble in life and in particular in, in quantum computing and the trouble comes for. Photonic, approaches, in the form of how. Do we answer this this question so, the flip side of these. Photons that don't interact with their environment, is that they don't interact with one another they don't interact with anything very, readily that's, the challenge and. This. Is the proof by cartoon, and at this point I'd like to do a survey and ask. If. You'd raise your hand if you've done this experiment. Okay. So that's that's for people which is which is a that's. An equal record that was just just. Set I think if Microsoft last. Week it's it's amazing how few people have. Done this experiment or are willing to admit that they've done this, experiment, right and I saw a few reluctant, hands raised there the. Reason is because, it's a pretty boring experiment, you know what's going to happen all those of you who haven't done it already know, what's going to happen nothing happens right. You. You. You you sort of should be a bit surprised by that if you think of it in terms of you know beams of particles flying. At the speed of light into one another but it does really does really. Tell you the issue. Here, in. Australia, where I come from this is what we call a backyard experiment. Because you go home from the lab at night and you do it in the backyard in the UK no one has a backyard and there's. A lot of talk around you know the state of science in the UK and I think this is that this is the real issue of, course here in sunny California, you guys have have plenty of space and backyards. And so on and that's, that's you know that's that's the reason for success I'm sure, okay. So that's the proof by cartoon, this is the sort of slightly more sophisticated, version. Of it imagine you wanted to realize a controlled-not gate so the quantum analog of an xor gate whether. Whether logical, operation on the two qubits is shown. There if. We had a path. Encoded. Target. Qubit where a, photon, in this top rail represented, a zero and in this bottom rail represented, a one we, could arrange an interferometer with.
A 50-50. Beam splitter here and a 50-50, beam splitter here which, both. Of those devices transmitted, half the light that comes in and reflect half the light that comes in and so, if we send a single photon in this, zero mode it, goes into a superposition of being in the top in, the zero mode and in, the one mode it then interferes, with itself in, such a way that it comes out here with certainty so there's constructive, interference ready to come out here and destructive. Interference for it to come out here and similarly. A single photon coming in this one input here, goes. Into the - superposition. Inside the interferometer and then, interferes with itself to come out the ones day, so. That's nothing more than classical. Interference of waves described. At the single photon level. And it's, also nothing, more. Than just the identity operation it doesn't do anything so, far it just Maps a 0 to a 0 and a 1 to a 1 and by, linearity of quantum mechanics, a superposition, of those two states at the same superposition. Which. Is precisely what you'd like to happen if you're in the top half of this table so, if you have your if. You have your control similarly, encoded, then very loosely speaking you want nothing to happen to, your control and if. The controls in the zero state you want nothing to happen to your target as I've just described, well, if your controls in the one state, you want, a bit flip operation, and the way you'd imagine doing, that potentially. Is to introduce a PI phase shift or 1/2 wavelength, change in optical path in one, arm of this interferometer let's, say the top arm conditional. On there being a single photon in the. Control one, mode and. So, that's that's job done that's how you realize a control. Not gate on on two photon qubits, the. Problem of course is that to, realize, this operation, here you'd have met you you'd imagine using some nonlinear, optical. Material, so a material, whose refractive, index depends, on the intensity of light in that material and what, you'd be requiring, is that the intensity of this one photon, here would, change the refractive index, such. That this other photon would see an effective pi phase shift and, it turns out that for conventional, nonlinear materials, you'd need something like 10 to the 9 meters of that, material for, the intensity of one photon to. Impart a pi phase shift on the other photon, now. I would argue that's not completely, impractical, you might imagine a spool of optical fiber that was 10 to the meters, long. That. Would say something about the clock rate of your computer, and, unfortunately. The transparency, of any such material is not is, not so high that you'd, have very much probability, of either of those photons coming out at the end and, so this was the dead end for for, optical. Quantum computing, at least as I'm I'm proposing, it here and. The, understanding, was you'd need some sort of exotic system, here like an atom cavity system that, would mediate an effective interaction, between, photons, and.
And. At. That stage you know. This. Was regarded as you know an interesting, photonics. Was interesting, in the context, of communication and an interesting, proving ground for. Quantum. Technologies, but not an ultimately scalable, approach until. Canole the flammond Millburn came, along and showed. That the intuition, that I've just sketched in some, laborious. Detail on the previous slide is wrong and, that in fact you can implement, that. Precise controlled-not, operation, on two photonic, qubits using only a linear, optical network so nothing fancy, in there just mirrors and beam splitters and so on, together. With auxiliary or, ancilla, photons. And photon. Detection, so. This this cartoon represents, a controlled-not gate in which you send in your your, control, and target photonic, qubits together. With some other photons. That don't encode any information, going in and when, you detect a single photon here and a single photon here out will come your, control and target photons, with. With. The appropriate, operation. Applied. To them now. This was a surprise, because. Of the intuition that I described. And in fact it was a surprise, to the authors so many can ill and Ray Laflamme set, out to prove the intuition, from the previous slide and as, as, sometimes happens, rather beautifully, in science they discovered, something far more interesting which is that that, intuition is wrong and the, opposite is true you can do it and they, went on to to. Present a. A. Recipe. For full-scale optical. Quantum computing using only the resources that you see on the slide here single, photons linear optical networks and single photon detectors. And I think at the time so. At that time I was working, on solid. State approaches, to quantum computing in particular phosphorus. And silicon. Approaches. And I think it was pretty fair. To say that this was received with okay well you know that's mathematically, proven to be possible but who really believes that you're going to make a computer, out of photons flying around at the speed of light and actually when you look at that the details the. Number of these auxilary, photons, that you that you'd actually need, okay. It was polynomial, and you get an exponential advantage, so you know if you're a theoretical, computer scientist that's fine its job done if. You're the guy who's got to build the stuff and that polynomial, is huge then that's pretty worrying and it was huge and, I'd. Say the, in the intervening you. Know 15 years or so the. The situation has changed and, in the first phase it changed you to theoretical, developments. Particularly. In, the realm of measurement, based or cluster state quantum, computing which reduced that overhead by many orders of magnitude and, then. In. More, recent times I would argue that all. Of the components, that you need have been demonstrated, in isolation, and in, conjunction with one, another in small, scale and there's a promise of actually being able to scale these things up and so, the. Summary situation, is if you want to pursue a photonic, quantum computer, there's, going to be a price to pay and you're going to need many more photons than then. You would need other physical, systems but, my argument is that's a small price to pay, relative, to the gain in scalability, and manufacturability which I'll talk about now. I won't, talk more about this, klm, scheme and I, should emphasize that I don't, think anyone really expects, to make a quantum computer pursuing. This type of. Klm, approach, circuit. Model approach but, it's useful from a pedagogical perspective, the. Reason that, people wouldn't pursue this approach is simply because the fault tolerance thresholds. That we know of for. Topological. Cluster state quantum computing is so good and there's not an expectation that they'll be matched in the gate model, okay. So I'll, just going to briefly explain to you what's going on in this, in this picture what what makes it work and then I'm going to start, moving fairly quickly through there through the technical, stuff.
So. What's going on inside there is quantum interference so. If you have a 50-50, beam, splitter as we encountered before and, we, send a single photon into. Each input as indicated, here such that they arrive at the beam splitter at the same time and we, ask ourselves what's. The probability, for one photon to come out the top and one, photon to, come out the right, and. If. We just naively looked at that picture classically, we would get the probability, of 1/2 because there's two ways for it to happen and we just use our usual probability. Theory but of course this. Is an inherently quantum mechanical, system and we should better, apply the, quantum approach to that which involves, summing, indistinguishable. Probability, amplitudes, which. Are complex numbers and can therefore interfere, in ways that their classical probability theorem, theory, counterparts, cannot, we. Take a mod squared to get sensible probabilities, between 0 & 1 at the end and what. Happens here is precisely that sort of interference because, we, get this phase shift on reflection and these. Two amplitudes completely. Cancel. One another, and. So. For me this is this is maybe. The simplest. Uniquely. Quantum mechanical, phenomenon, to, to. To understand, I have hopefully explained, it to you in just a couple of lines it's, also about as close as you can get to doing, quantum mechanics with your hands in that you can get into a lab and for example you can change. The arrival time of this photon with. Respect to this photon using, a micrometer to introduce. A delay for example and what you'll see is, data like this it's. Data that I'm showing you as a quarter of a century old. Nevertheless, graduate, students around the world still celebrate, when they see data like this because it's, it's, very hard to generate and, it's, hard to generate. Typically. Because in, writing, things down like this I'm implicitly, saying that these probability, amplitudes, are indistinguishable, from one another in principle. So. No measurement, allowed by the laws of physics could distinguish those two amplitudes, and as soon as that's as. Soon as that statement, is not true then this effect goes away. So. The reason that I've laid at the point is that this is all that's different in. Terms, of single. Photons than everything we know about bright, light, and it's this phenomenon, that drives. Drives, a quantum computer basically. Now. You'd like to know where where. The photons go and they're going into a coherent, superposition of, both being in this path and both being in this path and. If this beam. Splitter is not 50/50, then this, this, dip that we see here at zero, delay time doesn't, go all the way to zero but you still have a you.
Still Have a probability for that to work so for example here I have a 1/3 beam splitter, inside. What, is a linear optical controlled-not. Gate and. So again we've got our control and target modes and again we've got that interferometer, that we saw before and now. Our quantum interference as, I've just described between, a photon, in the control one mode and the, target photon imparts, that pi phase shift that we required. You. Can see directly that this gate doesn't work all the time a photon, could come out this top load or the bottom mode could get to here or to here it works precisely when, one. Photon comes out in the control and one photon comes out in the target, this. Is how my colleague Jeff pride and I first realized such, a gate in Andrew, White's lab. Using. These you know using, some tricks, on you. Know making interferometers, stable and so, forth you'll, note that in this circuit. There's, a control and target photon, going in and, I just told you that it works when I detect a photon here in a detector, photon here that's, not very useful right, in sight, of a computer, because detection. Of those photons, typically involves. Their destruction, and so, it's hard to embed that into a circuit, and so, a. Gate. Just. Like the original klm gate was some was, implemented, in them, she, gave taguchi's, lab in, a collaboration that we had going for for. Many years where you you you I mean, you can't probably do the translation, directly but it's this the. Control and target, photons, coming in and, these auxilary photons, which you then detect and then, your control and target photons, are then free propagating, afterwards again, this is this is not necessarily, the way you'd do it the, point I'd like to make here, is simply, that if, you saw that that. Circuit, in the lab or, or. These ones then they would all look pretty well the same and they, would look like a forest, of optical elements mirrors, beam splitter. Beamsplitters, and so unbolted, to a 1-ton vibration, isolation table. And this. This, skate here, might, consume. You know several square feet of table, space so if that's your transistor, you've got a very big computer at the end of the day and in. Fact. If. You know if you wanted to make a sensor, you know deployment. Is challenging, if it's bolted, to a 1-ton vibration, isolation table, and actually it takes really clever people like real aqua multi-and tom he's in Agartha you, know six, to eighteen months to get these things working. And you, know graduate. Students don't keep working for that long that you could imagine making circuits, you know many, times are more complicated than this and, that's what we've been working on at bristol over the last eight years or so the. First efforts were in we're in fiber which, I won't talk about I think they're important, in.
Terms Of communication, so making those same logic gates all in fiber but I think, in terms of ultimate scaling, you replace a forest, of optical elements with a bowl of spaghetti of, optical, fiber so the approach, that we have been pursuing, pursuing, is this you, know this cartoon, here of photons, in, wave guides on chip, and. I very much enjoyed, my um my, good friend and colleague Thaddeus, Ladd who was then at Stanford. Interrupting. Me when I first showed this picture at, a workshop in Tokyo, to say. Jeremy. Those wave guides they're very lossy, you know there's light scattering, out all over the place there I'm a little, worried about that and I said think thank you 30s for that question and view the low loss version, of the the artist's. Impression of the wave guides which he. Appreciated. The, idea is a simple one and that is to basically, fabricate. Square. Optical fibres on chip, so in this case in. Silica, on silicon. Where, you have a slightly higher, refractive. Index, core, of silicon with, with, a of, silica, with a slightly. Lower cladding. There any guide, light via total, internal reflection just. As in a fiber and if you make, the dimensions, right you can support just a single transverse mode. There then. You you make make. Your beam spitters by bringing two of these wave guides into proximity with one another such that the evanescent field, from, each waveguide couples to the other and by, controlling the length that coupling region you can control what the equivalent reflectivity, is. Could. Also do it by the spacing, between we use the length which is a more reliable way, to do it so. Using this approach we've implemented, that exact. Same scene update that I showed you before so control, and target modes here's that interferometer. Formed. By the two 50-50, beam splitters quantum interference at this central one and. The. Important. Point of this was that we saw that quantum interference that, I described, to you at the start with, a dip where, the visibility of that dip was, a hundred percent to within very small, error bars and that's important, because that would be a fundamental limit, to the performance. Of the operation, of these devices, we. Combined, several. Of these gates. Into a compiled, version of Shaw's factoring. Algorithm for factoring 15, and for. Those of you who don't know you should understand, compiled, not as everyone. Else would understand compiled, but, as a euphemism for already knowing the answer when you construct the circuit for the algorithm and I don't know how this abusive language propagated. But that's that's what it is so you. Inverted. Commas around compile, when you ever hear about a compiled, Shor's algorithm. We've. Been able to implement one qubit operations, with very high fidelity using, these these. Resistive, phase shifters, on on. The chip where you locally, heat the, waveguide, underneath and thereby change its refractive index we've, implemented one qubit operations, with 99.99, eight fidelity, I like to put that eight on the end there it's not quite five nines but it's sir it's, pretty close.
And. This. Technology is great. In every way it's very robust reliable repeatable etc, it's slow and and, that's a challenge that I'll come back to. We've. Also explored, just as an aside. Sort. Of models, outside of conventional, quantum. Computing so here's a device. Where, we implement a quantum walk and in this device you have 21 wave guides in this central region here instead. Of just two wave guides that are innocently, coupled you have twenty-one wave guides that are innocently coupled. With one another and you can implement some very, interesting quantum, walks there and it's possible that you might be able to directly do some, simulations, of important, physical, systems using that another. Application of that that I'll just mention in, brief because maybe you've, heard of this and you're interested this boson. Sampling, problem. Has, got quite a lot of some interest. Recently, and the idea is that if you take a. Unitary. Operation, for. Modes. For, optical modes let's say you have n, modes. And you send route. N photons, of, autoroute n photons into, those modes then, calculating. Where, those, photons, come out what the probability distribution, is for those photons is intractable on, a conventional computer and. I guess the reason that people are pretty excited by this is that once, you get of order a hundred modes and ten photons, that's, about the limits of what what my laptop could calculate and you don't have to go very much further before it's just simply not not-not-not. Calculator, ball I, won't. Talk about applications. Or lack thereof of that of that thing, I think it's pretty clearly an interesting thing to do to say you know I've got a device that. Will will, outperform, a classical. Computer and that's not not so far away of course, once. You cross that threshold where, you can't do you, can't use a conventional computer to tell you what the output should be how on earth do you verify the output of it and our. Approach here. Is to. Reprogram. Let let's say this unitary is reprogrammable which, I'll talk about in a little bit reprogram. It to implement the same unitary as that quantum walk would implement, and that is. Straightforwardly, calculate, of all what. What the output should be and, in fact what you see in those sorts of quantum walks is if you have three photons you see this sort of clouding. So this is the where, the photons come out with twenty one modes so on, each axis what you. Know each photon, in you see this sum is clouding behavior here in contrast to the classical behavior, where you see no. Such thing and. In you I can't really display four or five photon data, very easily but if you look at the the. Five photon data here then, you can see a clear contrast. Here so that's a that's a sort of verification at. A you know. An. Experimental, verification technique. I think which is quite interesting. But, what I'd really like to talk to you about is the problems and. How we've addressed them so this, is the this, is the chip that was used for the for the factoring algorithm and you, can see by inspection a few few problems here one, is scaling, so factors 15 and I I just admitted that it doesn't really factor 15, at all so how do we scale that up to do something useful, you. Can see it, doesn't have any knobs, or wires on it so it's. Not reprogrammable. To do other, things other than what it was fabricated to do and actually it's still too big right if that's a couple of transistors, then you still got a very very huge. Computer, at the end of it so, just, briefly how we've addressed those things I want. To just quickly, highlight that they're relevant in these other, scenarios. So I mentioned. This at the start this. Is a system. That we've that. We've now that this this one here that, we've now prototyped, and patented jointly with Nokia and to do that polarization, control, uses, exactly the same. Waveguide. Architectures, that I've described of course they're the challenges, to, make it for a few cents and fit into a very small bit. Of existing, chip ideally but but the, challenges, of that are the same in the sense that you need to make those components, very. Small and very highly. Functional, and again, I showed you this measuring. Of blood protein. Concentration. With, entangled photons and again a similar challenge if you want to deploy those sensors, where, you've got these microfluidic.
Channels And wave guides you need to you need to miniaturize them. In. A similar way and in fact for, anyone who's still interested in science and I certainly am. There. Are reasons for pursuing, these things to explore you know the very foundations, of quantum mechanics. For. Example, and this is an experiment where we've I think. We've shed some light on the, wave particle duality. Conundrum. That underpins. All of quantum physics in quantum, technologies. Anyway. But this is a this is a technical talk about, addressing, these issues and we've, addressed them in. The. Following ways so scaling you, know I'm a I'm a simple-minded. Experimental. Physicist, by training so I don't have grand ambitions of making exponential, improvements, in anything but, if I can make enough factor, of to improvement, I might be able to turn the, Impractical, into the practical and, this is an example of that I'd say so here's how you might implement, controlled. Unitary operations, and as, you'll no, doubt pretty. Well aware if you're if you're in this field all of all a quantum, computer really, does is to. Control. Unitary operations, a lot of very big controlled unitary operations, and so if we could do those more efficiently that would make life a lot easier, the, idea is trivial. Almost here's a control. Qubit. You could imagine as, many as you like and here are some target qubits and you, simply take your target. Qubits and based. On whether that control. Is in the zero-one you switch them into the red mode which bypasses the unitary or the blue mode which, uh experiences. The unitary so I hope you can just see immediately there that this does indeed do. The controlled unitary operation, where, that unitary, could, be a black box that I gave you where I didn't even tell you what that what the unitary was, and, that's. That's, quite a saving, over the usual decompositions. That you would do to, realize, the controlled, version of a particular unitary. And it's, it's applicable to any system where. You. Have access to these four, levels here, in a controllable, way and it's.
Precisely That that, circumvents. Are no-go theorem that you may be familiar with which suggests that you couldn't do this and you can't do this if you just stay in the qubit world. Um, we've, used that in a you know you know Shor's, factoring out of them factoring 21 the, bigger numbers not in the news the news is that we've done a sequence, of logic gates that, we've got a probability, distribution at, the end that's. Our non uniform, which is means. It's distinct, from noise. So. That's interesting. We've used it in a you know what I think is a more exciting. Simple. Application, and that is to implement, the phase estimation, algorithm which underpin. Shor's algorithm and a lot, of other important. Algorithms. And in this case we genuinely didn't know the phase before we before. We were in the olden so it's a small-scale algorithm, but it really calculates, something. And. I don't, really have time to talk about the details of this but we've also performed, well, we developed. A new algorithm for quantum, simulation for quantum chemistry, together with Alan aspera physics group at Harvard, and we've implemented that, on. A chip and that the headline, I guess, is that instead of doing, this sort of Trotter ization that you may be familiar. With where. You have a very very, long coherent. Operation, with, with many many gate sequences, the task is now simply to prepare States calculate. Expectation. Values of poly operators, on those, qubits your, Hamiltonian, is described, as a as a sum of you. Know simple products of those, poly, operators. And you can therefore efficiently. Calculate, from those expectation, values the energy of that state and then, you can use an a you can use a classical. Feedback. Loop. To then simply. Variational. E modify your. Input state and find, the ground state I think that's pretty exciting the. The, question, marks are over whether, the, measurement. Side, of things can be made for pull and so the distinction here is that the. Output is expectation, values of qubits which are you know it's not the usual sort of digital output. Anyway. On with this one, with this story so obviously, reconfigurability. Well you need lots of wires there's a bunch of wires going onto a chip those wires connect, to, these eight. Phase. Shifters here so you can see that, I've. Now got, these. Mark sender interferometers, but I've got a phase shift in the middle and afterwards, that allows me to send a single photon in this input for example and, prepare, any, one, qubit pure state at the output in principle here similarly, here you've, got the reverse over. Here and you've got one of these controlled-not, gates in, the middle and so. By. Setting those those. Phase shifters to a thousand, random values and then looking at the probability, distributions, at the output you can see how, robust, and reliable this, technology, is because the, fidelity is very nicely peaked, right new. One. You. Can, generate. Bell stage you can generate a sort of continuum, of entangled, States and perform a continuum, of Bell State type. Measurements. And you, can write sigh inside, the block sphere if. You trace over one of the qubits so you can prepare an arbitrary one qubit mixtape, and, at, this point we should have a raging, debate over whether size really the appropriate. Symbol to draw inside the block sphere I think, I'm willing to accept that ro would be far more appropriate. But I would also argue that size are much more beautiful character. To draw in there. If. You'd like to draw ro in inside, the block sphere then please go to this web address lot login to the device that I've that I've shown you control. Those phase shifters directly, yourself, and draw, this draw. There a symbol row with, it in all seriousness this, is available to anyone, with. Web access to. Log in and start programming. And using, this this small scale device and if you. Have serious, idea if you have any idea to do with it including serious ones and. You. Don't like the the GUI that has, you dialing up the the. Phase shifts you know with you with your mouse or whatever then let us know and you know you can plug directly into the Python script that runs it I mean it's sort of targeted, at school children and so, forth ok, miniaturization.
There's. A promising, one that's, architectural. And that is to replace those those, 2x2. Beam. Splitters within my end beams that is directly using a so-called multimode, interference, device and n-by-n. Beams that is a you know very. Important. Operation. And doing. Them directly instead of composing them into a whole lot of 2x2 themes, that is this is a great real, estate saving, on a chip and. Even greater real estate saving on the chip is to go from. This silica, devices, to, silicon, devices so, in all of these silicon, devices, that I've shown you the, size of the device is largely, dictated by, the, refractive, index contrast. Between. The core and the cladding which determines, how tightly the light is confined in those wave guides and that in turn tells you how fast you can go around the corner so just as if you take an optical fiber and you bend it enough the light comes out so, too if you go around the corner too fast with these wave, guides the light will spill out and the. Minimum Bend radius in all the devices that I've shown you so far is of order 10 millimeters, and that's why we have these relatively, large chips in these, silicon, devices here that minimum Bend radius is one micron, and so. The component, density increase, is. Then a million fold in going from these silica devices to silicon devices which, is a much bigger step in fact than going from the bench up to the first chip. Devices. Silicone, is appealing. For all sorts of reasons this, is this is just, just an aside that I like so here's your silicon waveguide your, single photons are indeed bright light propagating. Along here is some micro ring resonators that are coupled to that waveguide, with, a bragg grating, wrapped around the inside of it so, that the so that light is then emitted. Vertically, and this could be a solution, to chip stacking. With. Photonic, interconnects, or photonic vias between, them whether that's you know in the classical. Or quantum world and I think that's a really, important architectural, issue. Is you know how we get light into an orthogonal direction, but.
What I want. To spend a few minutes talking about now is the. Rest of the story so all I've talked about really so far is light photons. Flying around in the waveguides, on. A chip but we, of course have to get photons. In and out of there typically using. Fibers we have to generate those photons, typically. Using a process called spontaneous. Parametric down-conversion. Whereby. We send a bright laser beam into, a nonlinear, crystal such. That with. Very low probability one, of those photons, in the laser beams spontaneously, splits into two daughter photons, conserving, momentum and energy now. That's a very nice approximation, to two photons, and if you detect one of the photons you know that the other ones there with, certainty because, they're born in pairs but, it's totally useless in terms of making, a scalable, quantum computer, because it's spontaneous and. So you have no control over when that event happens or whether that event happens or not and. I'll come back to explaining. A solution to that in a moment, we of course detect, the photons using either semiconductor. Or superconducting, single photon detectors, do some sort of pre-processing. And, then, ideally we, feedback what-what, we learn on on, to the system itself and so, needless to say in the work that I've shown you so far the, whole quantum optics lab hasn't shrunk to the scale of a chip because of what all of this surrounding. Paraphernalia. Still fills it and what we'd like to do is head, towards this where, we have all of those components. Integrated. So. You know those nonlinear, sources, integrated, it's a single photon detectors, fast Reuters and so, forth and. Here's. A particular. Of what we'd like to do with that and that is a, mechanism, or, an. Architecture, for making, those non-deterministic. And. Therefore. Useless single photon sources into deterministic single, photon sources that, will do the job for full-scale. Quantum computing the, idea is a very simple one and that is that let's, say I have this source here I send my bright laser beam in.
I Send a pulse in. And there's. A low. Probability let's, say 10% of producing a pair of photons in that given, laser pulse and any other laser pulse if. I have any of those sources. All pumped with laser pulses, in parallel then the probability, of none of them producing. A pair of photons is negligible, right for any decent decent, end and. Then. My task is simply to take one. Of the seven or 12 or whatever it is per pulse of sources, that produced a pair of photons and. Detect. One. Of the pair which tells me with certainty that the other one of the pair is there and then, based on that. Use. This in by one switch. To switch that photon into this output and so. You've turned then. At least in cartoon form a you. Know a 1 or 10 gigahertz train, of laser pulses, into a 1 or 10 gigahertz train of single photons with, very high probability now. This. Looks like a brute force engineering, solution, to the problem and I totally. Agree that it is I also, think it's a very very promising one and it's promising, because we. Have very, nice control, over the photons we can generate beautiful. Photons in this process, dispersion, engineering, and phase matching engineering, allows, us to produce very. Nice photons, that interfere, with one another and is suitable for these, purposes, and then, furthermore. All of those things are sort of mass manufacturable. And and scalable, that's, that that's the argument that's not to say that there aren't plenty of engineering challenges in there but, once, you've got once. You've got you know a handful, of these things then scaling up to hundreds and thousands and so on which, should, be relatively straightforward, the. Final point is that once. You've solved all the problems of this you've in fact solved, all the problems for everything that you need for a full-scale quantum computer because that's that contains, all the elements that you need for, the aficionados, in the audience, this. Is your menu where you choose one of these approaches, for the sauces you choose one of these approaches, for the detectives, and so forth, I won't go into that in, any sort of detail but the point is that there are solutions, out there that have been demonstrated, I'll talk about some of the things that we've done and some of the things that other people have done is. A ring, resonators source, of photons. In in, silicon waveguides, we've looked at lithium tantalate and, also charcoal John I'd plenty, of people around the world have done all sorts of demonstrations, of generating. Photons in, nonlinear, wave guides on, chip the. Fast switches, for that that end by one Ruta that I showed you before, well I've, shown, you these thermal, phase shifters which are which it too slow and. In. The telecommunications. Well lithium, niobate, modulators. That operate at 40 gigahertz have been them have, been in in use for for. A long time and we've, used that same sort of technology to rapidly.
Manipulate. Paths and polarization, of single photons, in terms of detectors we really like these superconducting. Detectors. Which. Operate, at at. Around 3 K in, a closed cycle system, and that's, the only thing that's that's unfriendly. About them they have very low dark counts very low timing. Jitter in very. High efficiency. This. Is just a step. Towards, that vision of, multiplexing, where now I've got two sources on a chip with one another here and we, see quantum interference between, photons, generated in, those two, sources in this this. Beam splitter on the chip again, with. With. Unit visibility, or unit fidelity, to within very small error bars and I think that's a it's a very key step towards this you, know getting getting. You. Know hundreds of these sources running. In parallel. This. Is some work by other, groups showing those superconducting. Detectors, grown directly on gallium arsenide and silicon waveguides in the key point is very high efficiency. Because. You can mode match directly, to those detectives, this, is some work from Caltech showing them very. Long, low loss delay, lines which could be a very, very. Useful addition, to the, Vox, you'll, note that in the pictures that I've shown you got a bright laser beam coming in one side and single photon detectors sitting at the other side so you need a lot of attenuation, of that, laser beam you, know a hundred DB of attenuation and, again there's promising. Results out there for that and so. Back, to this cartoon, that I started with all. Of these elements have now been demonstrated. To the sort, of performance levels that are required in isolation, in some combinations, and now, the task is to you, know maintain the you know maintain. Those performance, levels as you integrate the, whole thing and manufacture. The whole thing to, give you a flavor of how the computation. Proceeds. Here's. The physical photons, in waveguides, and what, you do is you attempt, to fuse them into a cluster state and because, you don't have these deterministic, interactions, that. Fusion doesn't work with unit probability, and so you end up with a giant cluster state that has a lot of holes in it but, you're above a percolation, threshold which. Is which, is a phase transition so you know with certainty that, in some given volume you've, got you know in an, entangled, string of qubits, in each direction and, then, you essentially, renormalize, and say alright that block is my qubit and then i renormalize into a rouse and off lattice. And from, there for words it's exactly the same form. Of, fault-tolerant. Topological. Cluster state quantum computing as as anyone, who's pursuing a gate, model this is, looking at so the price that you pay photonic. Lis is that you've got this this additional. Step, here and then thereafter it's. The same now. The the, points, the final points to make well, firstly, show you how you might imagine doing that on this you know in a smaller cartoon so here's, a bunch of multiplex. Sources, ring, resonators, you. See four of them you should imagine four hundred of them and here's. Eight of them only for them are depicted but you can see there where. The other four would go you. Run that into a linear optical network, here and you, take your unentangled, single, photons and you, entangle, them into this star cluster state, here the success or otherwise of that circuit is is. Is. Given to you by the detection, pattern, that you get at the output here and then, you multiplex, this whole thing until you, you're, generating these, with, near, unit probability, and then, everything's. Ballistic, thereafter you simply, attempt. To fuse those things into the cluster state you, do that imperfectly but you're above a percolation. Threshold, through, that cluster and then you proceed as per here and you, get these you know the usual very nice fault. Tolerance thresholds. Probably. That there's this a couple of things to say and I'll. Start with the most important, thing, the. Physical. Depth so you imagine this computer, then looks like a. Bank, of single photon sources on one side and a bank of single photon detectors on the other and in between them is a is a big slice of this cluster state with, you know the dimensions. On the other in, the other direction is determined by the physical size of your computer the, length of the computation, that you do determine, by how long you run how, long you run this computation, for and the, point is that the, depth the physical depth between, the, sources and detectives, so the number of optical elements that they go through is fixed, as you. Scale up your computer, so the bigger you make your computer the. Depth of that thing remains, fixed that's. Very. Exciting. For me because if, I told you that you, know that depth grew even you know linearly, you'd, probably be a bit worried right because the bigger you make your computer the, more loss you're going to have them you know the more elements that you have to go through and that's simply a function of the other kind of you, know the the local operations, that you need to generate cluster, States so, that's that's very appealing the, other thing is that the.
Error, Model for. This. This, system is not yet known. But. There's good reasons to suspect that it might be quite, a bit more benign than the normal error models that we that we are used to and that is because of this, effective, zero temperature, that I described before so there's no intrinsic coupling, to a thermal bath so maybe those Pauli errors that we worry so much about usually. Are not applicable. There's. A prospect, to turn a lot, of errors, into, loss why. Would you want to do that well it turns out that loss is the best thing that can go wrong with your quantum computer you can lose in. You can lose 50, percent of of. Your, qubits and you can still do full-scale quantum, as, long as everything else works perfectly, so. If you so, if you can convert everything into loss then you have these incredibly, favorable, thresholds. And how might you do that well let's. Say you you have a polarization, encoded, cube head and it's depolarized, somehow well you put it through a polarizer you've turned that depolarization. Into loss let's say you've got some temporal, jitter in your photon while, you put it through a spectral. Filter. And you've. Turned that that, that. Timing, jitter into loss or if, you have fast enough detectors, you can indeed do that that filtering, temporally. So. There's great promise I think for turning these things into loss the only thing that I can think of that can't be turned into loss in that way which proves nothing I should add that just might, say something about my brain rather than about. Reality. Is. Dark. Counts on those detectors. Now. You. Might. Believe. That dark counters are more but you know it's a more benign error that you could deal with more. Directly and more efficiently than other sorts of errors and in fact it might be that you could, get it to this sort of you get dark counts to the ten to the minus twenty level where, you wouldn't encode against them at all. And. I. Think. That's that's, that's everything, that I that. I want to say about about. That. Approach. I think there's one more thing but it's escaped, me just, at the minute I've, probably said enough in any case and. Then of course you know this is the vision for you know stolen. Directly from IBM of of. Conventional. Computer chips in in the future where you replace copper, with, photonic.
Wires And so, with. A simple bit of Photoshop and relabeling, you just then have your photonic. Quantum computer, and all of the Associated, micro. Electronics, sitting right alongside and that's that's deliberately. A little bit facetious but, not completely facetious, and. So. I guess, just, I'll just finish by saying that, you know this is this is a way of seeing the the photonic quantum computing, Snack got all these components down here I'd, say that there's been plenty of work done down here plenty, of work done up up, here, and that the, tasks over the coming years is really to worry about things. In this in this middle region in. Sensitive architectures. And, there's plenty of plenty of interesting and exciting stuff. To be done. All. Right thank you. So. I would like to. Ask. You about two. Different things in one regarding. That detection, efficiency what. Are the practical limitations. Out the existing detectors. Especially. If you need to do photon, number. Resolution, also. The. Other question is regarding this the generation. Of the single, photon sources, I. Would. Like to see, what the scale. Up is with the that, kind of brute force method, that you mentioned, in, in in the sense of generating, a large number of single. Photons. At, the same time you know so making sure that it's you, have enough you, know time resolution, between, generation. Of those single photon sources choice, okay. So um, on. The detectors. There's, reports, of you know 95% efficiency. With these superconductors. And there's expectation. There may be even better already. In this expectation, that that, will get significantly, better it's, already well within the the. Threshold for this you know loss only, regime. That you'd like to work in I'm. Reasonably. Convinced. That our, task is to do. Sufficiently, compelling demonstrations. With those superconducting.
Detectors, That, you know a semiconductor. Company is sufficiently, inspired, to, then make semiconductor. Detectors, work. To the performance, levels required, ie. Put the tens or hundreds of minions into the problem that would. Be, needed. The. Reason they're not doing that now is that there's no market for single photon detectors, right quantum computing. Doesn't even rate a mention this. You know a lot, of biologists, doing microscopy and so on this you know there's not a big market for these things so. I think the detector thing again. There's, plenty of you, know important. And interesting engineering, to be done but it's it's it's definitely a solvable problem would, say and then the second question was about the, about. This sort of the, source. So the, the. Multiplex. Sources. And. This, is brute-force. Approach I, I guess the, promise of it is all about manufacturability. In the sense that you, know if you if you if you're making these these wave guides using. You, know a conventional, process if not exactly the same process that people in the semiconductor industry are using to make the, you. Know to make their optical interconnects, and make the you know that photonic layer that I showed you right at the end then. You know, once, you've got a few of these things working together, then scaling up should be should. Be reasonably straightforward. I've just shown you some you know sort of hot-off-the-press results. Of two, of those sources. Interfering. With one another and I'm pretty optimistic in, fact I've, advocated, to the guys back in Bristol why don't you just quickly make a circuit, where you have a hundred of these sources in, parallel, but, you only wire up three of them the first two and the last one and then, show me interference, between all three of those sources and I'll, be willing to believe that you know with high probability all, other sources in between well because you know I've used this you know this, reliable. Manufacturing, process to generate them they don't like the idea I think they want to wire up all hundred of them. Does. That answer your question. They. Don't quite get the point you set on these cluster. Vegetable a kelp one debilitation there's a good death dozen. So. The physical, death, doesn't. Grow and the. Actual circuit, depth so they're computation, that you're performing is just how long you run things for, how. Long you run the computation for see you you continue on one side you jet you're generating photons, at 10, or 100 gigahertz, let's say and then, you're entangling into the, slice of the cluster state and the clusters the actual you, know. Conceptual. Cluster state that you're doing the computation, on is the, physical dimensions, this way that determines, that and then in, this dimension, is how long you run the computation. For so it's, a physical depth so the number of elements that you have to go through and the point is that because, that's constant, that's. Just a clear fixed threshold that you have to get get, to no. Matter how big you want to build build, your computer in the other two dimensions which, terms the width of your computation. So you'd be a bit worried if I said you, know oh it's, you, know that the the physical depth grows you know, quadratically. With with, this size of the computation, then suddenly you know as the, computer as the computer. Gets bigger the, you, know I, you. Know the the probability, of actually exceeding, a threshold we you know decreases. That be set clear, well. I don't wait to get to still why, the. Basically. Why. The physical depth is fixed yeah, it's. It's simply because you, you know to generate, that cluster state just. Requires these some these. Local, kind, of interactions, so. Maybe. This is a this is a slightly clearer picture. Or maybe it's not, and. And. Here. You imagine, them, you. Know you you're. You're. Entangling, more, and more of these photons, on the right hand side and. It looks to be backwards this picture actually so, yeah so you you you're entangling more and more photons onto the right hand side and then you're measuring them out of the cluster on the left hand side so those ones you, should just ignore the great ones they measured out and so on and so.
To Actually generate that cluster state as you make the cluster state bigger in two dimensions, doesn't require any, greater, depth of two in terms of operation and then once you've got the cluster state all you're doing is doing measurements on a sheet at a time and feeding, the output on to the next one so. You, basically are measuring the cluster state asset is be exactly. Streaming, the class exactly yeah, so, you don't you never have to you never have to see the whole cost to state in existence, if you're just you. Know. So, I I, guess. None. Of the components, scare, me. What. What, what's, most challenging where there's most work to be done is in that multiplex that sources us as I say and I you know the state of the art is you know, interfering. A couple of sources but the point is that you, know doing that in a you, know using, scalar scalable. Technology to make them holds. Promise so, that um, that. Worries me a bit but I think the thing that really worries me is the kind of assembly and manufacturer. Off the wafer so, likely. This you know billion cubic, device doesn't fit on a single wafer. My. Back, of the envelope calculation. Suggests that, you, know you might need, something in you know that this that. This thing here. Well. This this thing might, be sort of you know but, this kind of dimension. In cross-section so you've got you know a bank of single, photon detectors here running through and. Detectives. At the other end. So. Making of making. A photonic. Brick. If you like. That. That, doesn't. Scare me it excites, me but it's, that you know it's not been done before, and, it's beyond what you. Know what the semiconductor, industry might, do and assembling, that thing optically, electrically, and mechanically. It. Represents. Outstanding, challenges, but I guess the point is that all, the bits and pieces themselves, we can stamp out from on. Silicon, wafers. And I, think, it. Would be exciting to understand. Just, what you could do on on a single, wafer you know like how biggest. Scale could you get to and could you take some some. Problem, and and. Really, perform that on there you know so I think. They've been in qubit devices, that, say in 24-bit. Factoring. Machine so, you know basically. A reprogram. Of all digital quantum computer but, I think there are great prospects, for smaller, scale devices that attack particular problems, very efficiently, and where. There's a sort of a fit to the app a fit, of the algorithm to the hardware if you like at the hardware of photonics. And. I think this this some boson, sampling, whilst, isn't, you, know it isn't clear that the there. Is or ever will be a an application, for it it's a very nice example of fit, to the hardware right because that thing does work deterministically. You know you've got non interacting bosons just, launched photons, into this thing and ask the photons to do what they naturally want to do you know do what do what you do best just fire through that thing and you've got an example of things now I think, all, you. Know c
2019-03-18 23:24
Jeremy I hope it (last linck) will convince you I was right every time, excluding small errors , any wanted, any because I send everything without to re see because if you re-maind 3 years before this will same to be a foolish thing and isn't. #HumanRights #copyright #HumanityScienceThesaurus #AmericanScienceThesaurus #EuropeanScenceThesaurus #EarthScienceThesaurus #Inertia #NumberPhile #TheTearOfGeea #ClimaticHarmony #TheWheelOfLife #IQ #Nobel #UNESCO #Math #Mathematica #Stiinta #Matematica #quotes #Rieman Down here after an play on errors you will get the Real solution for Fermat's Last Theorem and a new maybe Fermat Equation , and a lot more. FERMAT WAS GENIAL WITH ITS EQUATION - THERE ARE A LOT OF ASPECTS WHICH CAN BRING A LOT IN SCIENCE. I love our Space Adventure but in the Same time we need to not forgot two things. 1.) we not all people will can go on Mars or Elsewhere 2.) after 60 years or more of Space Adventure we are not yet in the point we dreamed then. Then we need to Stand up for our Ethic and moral principles, nobody is perfect but need to try to respect each other because we have yet a lot of work to make. Also we need to have a inestimable respect for Earth resources at the last until our Space Adventure will add any efficiency. - Our primordial Duty is to push The Life to Infinity and Human Life maybe in Special because is one of intelligent life what to can do it.- I said to you long time ago #InMurguCUTandPT345Resonator have a big base even in Abstract. Human Rights, Even if My name is "Disorder" my work will bring a lot even in Math: 1) Ion Murgu Circles Paradox 2) Ion Murgu Divegent Conjectures unfinished yet maybe 3) Ion Murgu Powers Algebraic Triangles 4) Ion Murgu Infinity Exact numbers Generators. Fermat's Last theorem was not My direct scope now I think a pure Mathematical Method to solve Fermat stand up into 3 equations X^2 +Y^2 =Z^2 ,KX^2 +KY^2=KZ^2 , (KX)^2 +(KY)^2=(KZ)^2 - the Future will decide , but My Scope was (Z-1)^n+ (Z-2)^n = Z^n which can define the apparently points of conjecture relatively to n and into Integers is valid only for n =2 and My Team from #IonMurguCUTandPT345Resonator which I think define maybe first Law of Matter conservation. See now it without errors. Now Complete, second important Property For Team(3,4,5) (sorry for mistaking but was a need) . Ion Murgu Powers Algebraic Triangles & Fermat's Last Theorem End. I think for everybody now is evident this is the single solution which to not send Physics, Chemistry and Math in a wrong direction, anyway is coming back with a lot a new nice problems and maybe more interesting aspects for all area there. Bringing Fermat's Last Theorem at form (Z-1)^n + (Z-2)^n = Z^n FALSE this false equation for every n will have its role into the future. Only for Team 3,4,5 Truth. This not only Solved Fermat in an correct Mode, but is bringing a lot for Future. this Equation can be proved False now using more methods and imply X^n + Y^n =Z^n into integers False. Ended this problem Natural and Clear and I hope everybody already intuited next Fermat problem in there. "http://www.climaticdisorder.com/FLT-End/FLT-Second%20end.htm"
+Ion Murgu Dude, WTF
Jeremy O'Brien: "Quantum Technologies"
I understood some of these words!
this can only take place as an April Fool. I love the design of the slides, seems to come from the Future Past os, Windows 95. Show me the way, you poor gods wannabe.
Tl;dr version. 21m0s pretty much sums it all up when talking about the beam splitter. "50% BS". That's how to summarize the latest advancements in quantum. Everything including the D-Wave is roughly 50% BS ;)
The first experimental realization of a CNOT gate was accomplished in 1995. Here, a single Beryllium ion in a trap was used. The two qubits were encoded into an optical state and into the vibrational state of the ion within the trap. At the time of the experiment, the reliability of the CNOT-operation was measured to be on the order of 90%. In addition to a regular controlled NOT gate, one could construct a function-controlled NOT gate, which accepts an arbitrary number n+1 of qubits as input, where n+1 is greater than or equal to 2 (a quantum register). This gate flips the last qubit of the register if and only if a built-in function, with the first n qubits as input, returns a 1. The function-controlled NOT gate is an essential element of the Deutsch-Jozsa algorithm. CNOT is also a kind of universal gate(in the classical sense of the word). It is easy to see that if the CONTROL is set to '1' the TARGET output is always NOT. So, a NOT GATE can be constructed using CNOT.
sorry to repeat I love Entanglement , but is Quantum Wave asymmetry and Quantum wave regenerations, ok you don't trust my analyze , but let it for the future too, two way in comparision is the best to not make an error in lopsing the road